4.6.4: Power Reactors
- Page ID
- 85100
It is possible to build high-power reactors using natural uranium as the fuel, and heavy water \( D_{2}O \) as the moderator – as discussed in the preceding section. This design is used in Canadian CANDU reactors – there are some such units in power plants generating as much as nearly 1000 MW of electric power. Natural uranium is the cheapest reactor fuel – but, regretfully, \(D_{2}O \) is pretty expensive and therefore the CANDU-type reactors have never become very popular – there are only about 30 of them inactive service worldwide, out of the total number of 449 ((data for the end of the year 2017).
Another option for natural uranium rectors is to use high-purity graphite as the moderator, much less expensive than \( D_{2}O \). In fact, all reactors built in the US during the first few years of the “Atomic Age” were graphite moderated. Such reactors are perhaps the best choice for the production of Plutonium, the material needed for making nuclear weapon. But definitely they are a poorer choice for civilian electric power generating plants. Such reactors are known to be unstable in certain circumstances. An instability resulting from human errors led to the catastrophic explosion of the huge RBMK-1000 graphite power reactor at Chernobyl in 1986. In 2013, there were still 11 such reactors in service in Russia, but new units have not been built after 1986.
The most common type now is a reactor moderated by ordinary water, \( H_{2}O \). From the discussion following the derivation of the Eq. 4.8 one might conclude that ordinary hydrogen, with its nucleus consisting of a single proton, should be even a better moderator than Deuterium whose nucleus is approximately twice as heavy as a single proton. This is true – a neutron loses more energy in a collision with a single proton than with a deuteron. But protons not only slow down neutrons – quite often a neutron hitting a proton “decides” not to get bounced off, but to stay coupled with it instead. Such a neutron, of course, is lost and cannot support the chain reaction. In other words, ordinary water has too strong an absorption coefficient: there is no way of building a natural-uranium fueled reactor with a \( H_{2}O \) moderator. Yes, natural-uranium fueled. But once “enriched” fuel became available – i.e., a mixture of uranium isotopes with the U-235 content significantly higher than 0.7% – building reactors moderated with ordinary water became feasible. It turns out that enriched uranium with U-235 content of 3-5% already makes a good fuel for such reactors.
Today, over 400 power reactors operating worldwide are water-moderated. There are two basic types used, “Boiling Water Reactor” (BWR) and “Pres- surized Water Reactor” (PWR). The basic design scheme of each type is shown in Figs. Figure \(\PageIndex{1}\) and Figure \(\PageIndex{2}\). In both types water is used both as a moderator and as a coolant. In both types the fuel rod assembly (the reactor’s “core”) is placed inside a pressurized water tank, usually referred to as a “vessel”. The BWR design is simpler. It operates at a pressure of about 7.5 MPa ( 75 times the atmospheric pressure) at which the water boiling point is close to 300◦C (the dependence of the water boiling point on pressure is shown in Fig. Figure \(\PageIndex{1}\)). The thermal power generated in the core heats the water to the boiling point, and the steam generated by the process is sent directly to a turbine – where its thermal power is converted to mechanical power, which is used next to operate an electric generator. The exhaust steam is next sent to a “condenser”, where it’s cooled down and changed back to water – and then injected back into the pressure vessel by special pumps.
One disadvantage of the BWR system is that water/steam which inside the reactor is in direct contact with high radiation is sent directly to the turbine – the water becomes radioactive and even though its radioactivity decays in days, a leak in the steam/water loop may cause a serious safety hazard.


A much safer design is the PWR reactor in which water that is in direct contact with the core travels only in a closed “primary loop”. The pressure in the reactor vessel is kept higher than the water boiling point, so that it always stays liquid. An essential component of the primary loop is a “steam generator” – essentially, it is a special type of a heat exchanger, a device used to transfer heat between two streams of gas or liquid.
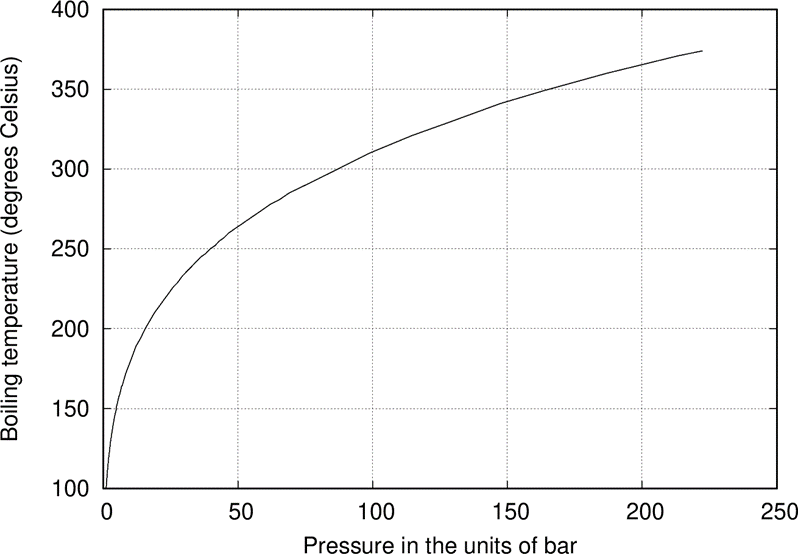
The primary loop water is pumped through the generator, where it passes its thermal energy to a stream of water from the secondary loop. The water from the primary loop and that from the secondary loop are not in direct contact – all heat is passed from one stream to the other through the walls of metal tubes. Before exiting the generator, the water in the secondary loop is hot enough to change to steam. This steam is sent to a turbine, then the exhaust steam is condensed to water and sent back to the steam generator. So, the steam that is operating the turbine never gets in contact with high radioactivity. The PWR design is thus safer that the BWR one – on the other hand, it’s more complicated and its efficiency of converting heat to mechanical power is slightly lower than in the case of the BWR reactors.
It should be noted that both BWR and PWR reactors have an “intrinsic safety mechanism” built in: if the cooling system fails and the water leaks out, the chain reaction in the core simply stops. It’s not the end of the problems because the decay of the short-lived fission fragments still generated heat and after a short time the core may start melting and shortly afterwards may form a “pool” of molten metal at the bottom of the vessel. But at least there is no such an explosion as the one that happened in Chernobyl: in a graphite reactor, cooling water is circulated through a network of pipes inside the graphite core – but it is the graphite which is the moderator. So, If the water leaks out, the chain reaction does not stop – in a moment the reactor may get catastrophically overheated and explode.
Over the years, there has been a steady progress in developing new de- signs of BWR and PWR reactors with improved parameters and enhanced safety systems. The reactors built before the year 1990 are referred to as the “Second Generation” devices, and those built later, as the “Third Genera- tion” ones. Now, most attention is attracted by “Generation IV” reactors which have not yet been built, but “are on the horizon”.