3.6: Current Flow in Two Terminal Quantum Dot/Single Molecule Devices
- Page ID
- 50028
In this section we present a simplified model for conduction through a molecule. It is based on the "toy model" of Datta, et al.\(^{†}\) which despite its relative simplicity describes many of the essential features of single molecule current-voltage characteristics.
The contact/molecule/contact system at equilibrium is shown in Figure 3.6.1. At equilibrium, \(\mu_{1}=E_{F}=\mu_{2}\). Since there are two contacts, this is an example of a two terminal device. In keeping with convention, we will label the electron injecting contact, the source, and the electron accepting contact, the drain. We will model the molecule by a quantum dot. This is accurate if the center of the molecule is much more conductive than its connections to the contacts.
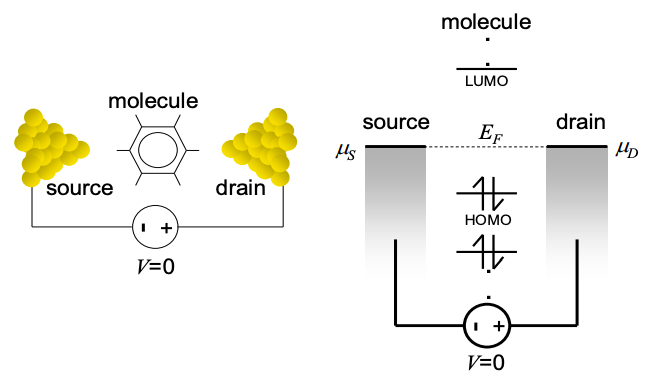
Now, when we apply a potential between the source and drain contacts we shift Fermi level of one contact with respect to the other, i.e.
\[ \mu_{D}-\mu_{S} = -qV_{DS} \nonumber \]
There are two effects on the molecule:
- The electrostatic effect: the potential at the molecule is changed by the electric field established between the contacts. The energy levels within the molecule move rigidly up or down relative to the contacts.
- The charging effect: Out of equilibrium, a current will flow and the amount of charge on the molecule changes. It may increase if current flows through the LUMO, or decrease if current flows through the HOMO.
Unfortunately, these effects are linked: moving the molecular energy levels with respect to the contact energy levels changes the amount of charge supplied to the molecule by the contacts. But the charging energy associated with charge transfer in turn changes the potential of the molecule.
We will first consider static and charging effects independently.
Electrostatics: The Capacitive Divider Model of Potential
Our two terminal device can be modeled by a quantum dot linked to the source and drain contacts by two capacitors, \(C_{S}\) and \(C_{D}\), respectively. The values of these capacitors depend on the geometry of the device. If the molecule is equi-spaced between the contacts we might expect that \(C_{S} ~ C_{D}\). On the other hand, if the molecule is closely attached to the source but far from the drain, we might expect \(C_{S} \gg C_{D}\). (Recall that the capacitance of a simple parallel plate capacitor is inversely proportional to the spacing between the plates.)
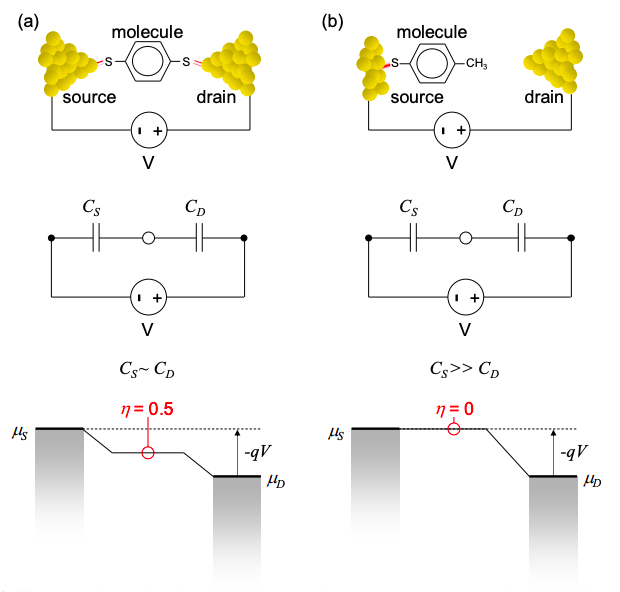
These two potential profiles are shown in Figure 3.6.2. The voltage is calculated from the capacitive divider. Thus, an applied voltage, V, shifts the chemical potentials of both the source and drain contacts:\(^{†}\)
\[ E_{F}= -\frac{1/C_{S}}{1/C_{D}+1/C_{S}}qV_{DS}+\mu_{S} \nonumber \]
It is convenient to use the Fermi energy of the molecule at equilibrium as a reference, i.e. if we set \(E_{F} = 0\):
\[ \mu_{S}= +\frac{C_{D}}{C_{S}+C_{D}} qV_{DS} \nonumber \]
\( \mu_{D}= -\frac{C_{S}}{C_{S}+C_{D}} qV_{DS}\)
We can define a voltage division factor, \(\eta\).\(^{†}\) It gives the fraction of the applied bias that is dropped between the molecule and the source contact, i.e.
\[ \eta=\frac{C_{D}}{C_{S}+C_{D}} \nonumber \]
As shown in Figure 3.6.3, the voltage division factor determines in part whether conduction occurs through the HOMO or the LUMO. If \(\eta=0\), then the molecular energy levels are fixed with respect to the source contact. As the potential of the drain is increased, conduction eventually occurs through the HOMO. But if the potential of the drain is decreased, conduction can occur through the LUMO. The current-voltage characteristic of this device will exhibit a gap around zero bias that corresponds to the HOMO-LUMO gap.\(^{†}\)
If \(\eta=0.5\), however, then irrespective of whether the bias is positive or negative, current always flows through the molecular energy level closest to the Fermi energy. In this situation, which is believed to correspond to most single molecule measurements,\(^{1}\) the gap around zero bias is not the HOMO-LUMO gap, but, in this example, four times the Fermi energy – HOMO separation.\(^{§}\)
The voltage division factor is a crude model of the potential profile, which more generally could be obtained from Poisson‟s equation. \(\eta\) is also likely to vary with bias. At high biases, there may be significant charge redistribution within the molecule, leading to a change in \(\eta\).\(^{†}\)
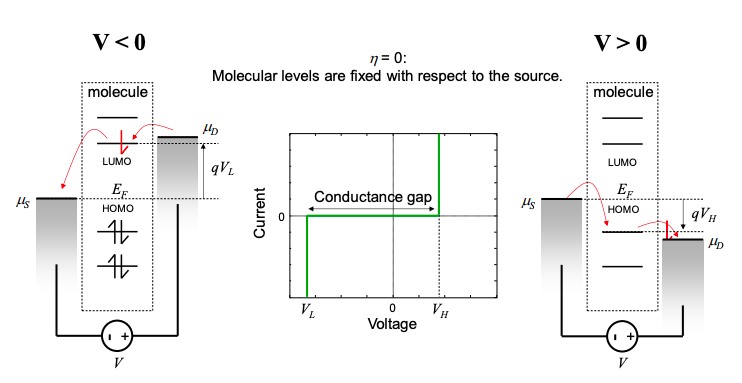
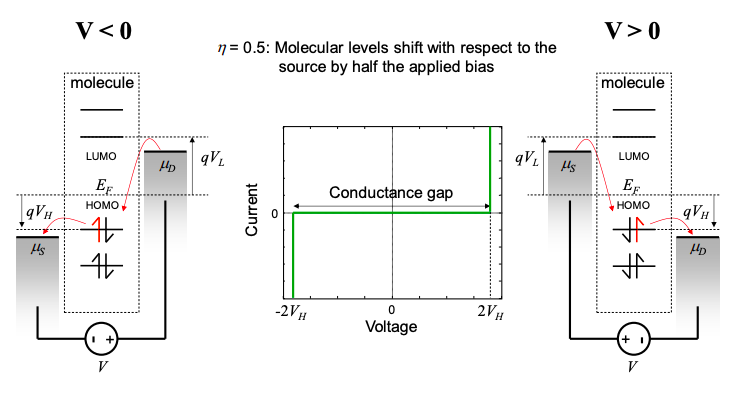
Charging
Previously, we defined the charging energy as the change in the molecule‟s potential per additional electron. To calculate the net effect of charging we need the number of electrons transferred.
At equilibrium, the number of electrons on the molecule is determined by its Fermi energy.
\[ N_{0}=\int^{\infty}_{-\infty} g(E)f(E,E_{F})dE \nonumber \]
Under bias, the electron distribution on the molecule is no longer in equilibrium. We will define the number of electrons under bias as N.
Thus, the change in potential at the molecule due to charging is
\[ U_{C} = \frac{q^{2}}{C_{ES}}(N-N_{0}) \nonumber \]
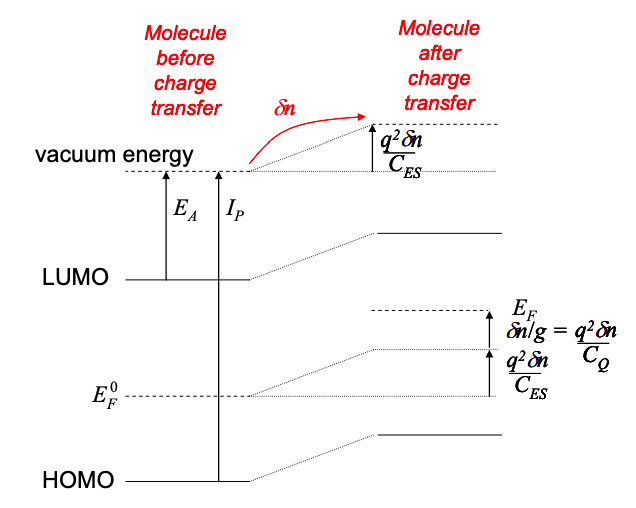
Summary
The net change in potential at the molecule, U, is the sum of electrostatic and charging effects:
\[ U=U_{ES}+U_{C} \nonumber \]
By applying the source-drain voltage relative to a ground at the molecule we have forced \(U_{ES} = 0\) in Figure 3.6.4. But it will not always be possible to ignore electrostatic effects on U if the ground is positioned elsewhere. Analyses of transistors, for example, typically define the source to be ground.
We model the effect of the change in potential by rigidly shifting all the energy levels within the molecule, i.e.
\[ g \rightarrow g(E-U) \nonumber \]
\(^{†}\) S. Datta, "Quantum transport: atom to transistor" Cambridge University Press (2005). F. Zahid, M. Paulsson, and S. Datta, "Electrical conduction in molecules‟. In Advanced Semiconductors and Organic Nanotechniques, ed. H. Korkoc. Academic Press (2003).
\(^{†}\) F. Zahid, M. Paulsson, and S. Datta, "Electrical conduction in molecules". In Advanced Semiconductors and Organic Nanotechniques, ed. H. Korkoc. Academic Press (2003).
\(^{§}\) It is possible to experimentally distinguish between \(\eta=0.5\) and \(\eta=0\) by choosing contact metals with different work functions. If the conductance gap is observed to change then it cannot be determined by the HOMO-LUMO gap, and hence \(\eta \neq 0\)