6.3: Molecular orbitals
- Page ID
- 50037
Unfortunately, even when we apply the Born-Oppenheimer approximation and hold the nuclear coordinates fixed, the solution to the Schrödinger equation (Equation (6.2.5)) is extremely complex in all but the simplest molecules. Usually numerical methods are preferred. But some conceptual insight may be gained by assuming that the molecular orbitals are linear combinations of atomic orbitals, i.e., we write:
\[ \varphi = \sum_{r} c_{r}\phi_{r} \nonumber \]
where \(\varphi\) is the molecular orbital and \(\phi\) is an atomic orbital. A filled molecular orbital with lower energy than the constituent atomic orbitals stabilizes the molecule and is known as a chemical bond.
We can define two types of molecular orbitals built from s and p atomic orbitals:
\(\sigma\) molecular orbitals: These are localized between atoms and are invariant with respect to rotations about the internuclear axis. If we can take the x-axis as the internuclear axis, then both s and \(p_{x}\) atomic orbitals can participate in \(sigma\) molecular orbitals. \(p_{y}\) and \(p_{z}\) atomic orbitals cannot contribute to s molecular orbitals because they each have zero probability density on the x-axis.
\(\pi\) molecular orbitals: Electrons in \(\pi\) molecular orbitals are more easily shared between atoms. The probability density is not as localized as in a \(\sigma\) molecular orbital. A \(\pi\) molecular orbital is also not invariant with respect to rotations about the internuclear axis. linear combinations of \(p_{y}\) and \(p_{z}\) atomic orbitals form \(\pi\) molecular orbitals.
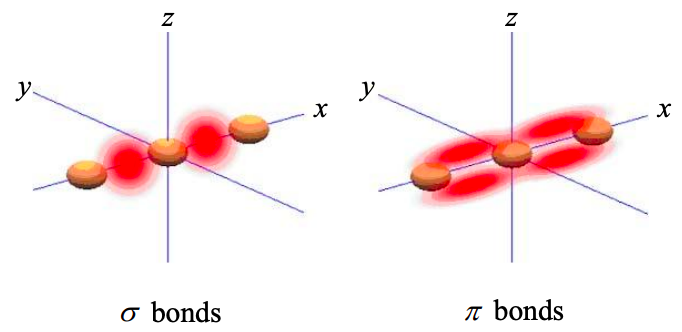