6.3: Applications- Land and Off-shore Use
( \newcommand{\kernel}{\mathrm{null}\,}\)
It is not known exactly when people started using the power of wind to do work on land – work which previously needed engaging human or ani- mal muscles. Probably it was not as early as harnessing wind for propelling riverboats. The latter requires using a big piece of cloth and a few poles and pieces of rope. Performing land works such as grain grinding or water pumping – which were the first task wind was used for – already required building a machinery. There are indications that Hammurabi, the king of Mesopotamia, tried to use windpower for irrigation purposes as early as 1800 B.C.. But harnessing wind power at a larger scale has began only in the Modern Era (see the document History of wind power development . The first known wind machines were vertical-axis windmills built in Persia (today’s Iran) and Afghanistan. It seems that the art of building windmill was brought to Europe from Middle East only towards the end of the Medieval Age. The Persian-type vertical axis windmills were soon replaced by more efficient horizontal-axis models, the precursors of modern wind turbines. The progress in windmill technology was the fastest in the Netherlands where such machines were crucial for pumping water from fields which otherwise would be flooded by seawater. In other countries the principal task of windmills was rather grain grinding. Windmills competed with mills using waterwheels and were certainly victorious in areas where creeks were scarce but there was much wind. Windmills, for instance, were popular in Spain – but not only for grain grinding, as is described in the literary masterpiece Don Quixote novel (see the article in Encyclopedia Britannica) written by Miguel de Cervantes in 1605.
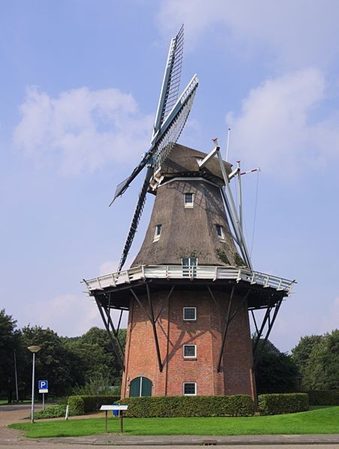
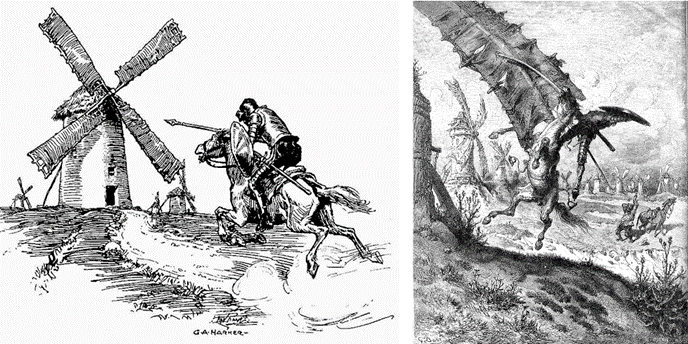
A highly successful windmill type was the American multi- blade wind turbine. The manufacturing of early models with wooden blades started around 1850. No long afterwards they were replaced by steel profiled blades. The main application of the turbines was water pumping. The turbines were manufactured for over one hundred years. About six million of them were installed all over the United States, mostly at farms. A large American turbine-type rotor was used in 1888 in a pioneer installation for generating electricity built in Cleveland, Ohio by Charles F. Brush (see Fig. 6.13). It generated 12 kW of electric power and worked for the next twenty years.

However, in general towards the end of the XIX century the use of wind- power started dwindling because of the emergence of steam engine and later, electric motors and Diesel engines. Research on possible new applications of windpower did continue, but not at a large scale.
A renaissance of interest in windpower was triggered by the Oil Crisis of October 1973, when the oil prices surged overnight from $3 to $12 for a barrel due to embargo for selling petroleum products to the US imposed by OPEC countries. From today’s perspective $12 may seem as a bargain, but in 1973 it was a tremendous shock. All of a sudden methods of generating power not involving combustive materials started attracting considerable attention. It coincided with environmental concerns that scientists had started raising about a decade earlier. At the time of the Oil Crisis scientists’ concerns were already shared with a broader public opinion. The atmosphere was therefore conducive for launching new research programs on “clean energy”. In particular, research sites where scientist and engineers developed and tested new types of wind turbines capable of generating electric power mushroomed all over the US and Western Europe. The goal was to find designs that would combine several advantages, such as: high efficiency and high output, sturdiness warrantying long periods of reliable action with minimum maintenance servicing, easiness of mass production and of setting up “wind farms” grouping large numbers of individual units.
There were many competing ideas. For example, some engineers opted for turbines with vertical axes – like in the old Persian windmills – but with highly sophisticated rotors. Such machines, it was argued, offer two considerable advantages: first, the electric generator may be then placet on the ground level. It makes servicing it much easier – and the column, carrying only the rotor, may be much lighter. Second, a vertical-axis rotor works well no matter from where the wind blows, there is no need for adjusting anything if the wind direction suddenly changes.
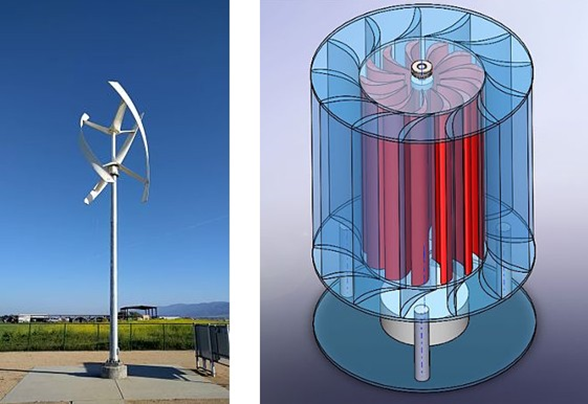
The alternative is a turbine with a horizontal axis, reminiscent of the old Dutch windmills. Here, there is a dilemma: the most straightforward option is to couple the electric generator to the same shaft to which the rotor is attached – meaning that the tower should carry both the rotor’s and the generator’s weight. Or, one can “transmit” the torque from the rotor to the ground level by a long shaft running inside the column by applying a 90◦ gear at the top of the tower (which, in fact, is the case in the Dutch windmills). Another disadvantage of the “Dutch style” is that the turbine rotor must always face the incoming wind – which requires installing an extra wind direction sensor, and a mechanism that would turn the entire “tower head” if the wind direction changes.
It should be noted that analogous situations happened many times before in the history of technology. Take, for example, the automobiles. At 1910 there were three types of cars available in the US. The largest group were electric cars. There were also gasoline cars, and there were steam cars (yes!1). But finally, after about 1925, the gasoline cars were the winner, and the other two types simply disappeared. What decided about the gasoline engine victory? Well, a whole bunch of entangled factors – not only of technical nature, “marker forces” also played a significant role.
It is difficult to say what exactly decided about the winner in the competition between the many wind turbine designs. There were too many quarrels, failed tests, successful tests, bankruptcies, etc. – too many even for trying to present a very brief account of what happened during the two tumultuous decades in the windpower R&D and marketing sectors after the Oil Crisis of 1973 – but a good review of important facts is given in Parts 3 to 5 of the Illustrated History of Wind Power Development.
In short: it is clear who is the winner. Take a ride over rural regions of the United States where there are many windfarms. Or a trip over rural Germany (over there, one doesn’t need to look for a windfarm – it is difficult to find a spot in that country from which it is not possible to see any wind turbine). Everywhere you go, you see the same standard model: a horizontal- axis three-blade turbine with the electric generator positioned right behind it, in a “nacelle” situated at the top of a tower. The turbine should always face the wind, i.e., the turbine axis should always be parallel to the wind velocity vector. The misalignment between these two directions is called the yaw angle – for ideal turbine work, this angle should be zero. If the wind direction varies, the nacelle can be rotated in a controlled manner in order to always keep the yaw angle possibly close to zero.
In YouTube, one can find good videos explaining how different elements and mechanism work:
- How the blades are profiled and how the turbine “pitch” is controlled:
- How the wind direction and speed are determined by the on-board electronics, and how the nacelle yaw and the pitch of the blades are varied by small electric motors with appropriate gears depending on the wind direction and speed;
- How the electric generator is coupled with the turbine.

The rotor of large “wind power converters” – as the manufacturers prefer to call their products – do not turn very fast: typically, 15-20 revolutions per minute (rpm). Most often, the electric generators used have to turn much faster, 1500-2000 rpm. In such designs the rotor and the generator are connected via a gear that increases as much as needed the “output” speed of rotation. The “anatomy” of such a model is shown in this YouTube clip.
Another possible solution is to use a generator turning with the same speed as the rotor – it’s called a “direct drive”. The generators used in such arrangements, called annular (meaning “ring-shaped”) are of large diameters. A process of manufacturing a generator of such type is shown (starting 7 min. 45 s from the beginning) in this video made by Enercon, a major manufacturer of high-power wind energy converters.
The most powerful wind power converter – as of the end of 2017 – is the 9.5 MW offshore windmill shown in Fig. 6.3.5. The list of 10 most powerful wind machines, periodically updated, is given by the Windpower Monthly magazine. The largest on-land wind machine is the 7.5 MW Enercon E-126.

The Windpower Monthly magazine provides a list of top ten wind turbine makers in the world. The list of all current manufacturers worldwide can be found at the Web page of The Windpower - Wind Energy Market Inteligence2. By clicking on the manufacturers name, one can get for free the list of wind turbine models ever made by this company. There is much more interesting information i n the latter Web site, but – unfortunately – one has to pay for it. The total installed windpower nametag capacity3 worldwide approaches 500 GW (as for the beginning of 2018). As can be seen in Fig. 6.13, the growth is exponential-like, with the total capacity doubling over a period of about 5 years. One can expect that the 1000 GW level will be reached about the year 2023 (for comparison, the total installed nametag electricity generation capacity in the United States, according to the U.S. Energy Information Administration, was 1177 GW in 2016, and the total windpower nametag generation capacity was in the same year was 82 GW).
_______________________________________________
1. The famous Stanley Steamer vehicles did not look like a railway steam engine: no smokestack, no towed coal-carrying tender. They were elegant cars, using gasoline as the fuel, looking even better than other car types of the same era.
2. Not the same organization as the Windpower Monthly.
3. Nametag capacity – a.k.a. the nominal capacity, rated capacity, installed capacity, maximum effect, is the maximum possible sustained output from a facility, e.g., from a power generating plant.