10.3.2: Biodiesel Fuel
- Page ID
- 85158
\( \newcommand{\vecs}[1]{\overset { \scriptstyle \rightharpoonup} {\mathbf{#1}} } \)
\( \newcommand{\vecd}[1]{\overset{-\!-\!\rightharpoonup}{\vphantom{a}\smash {#1}}} \)
\( \newcommand{\id}{\mathrm{id}}\) \( \newcommand{\Span}{\mathrm{span}}\)
( \newcommand{\kernel}{\mathrm{null}\,}\) \( \newcommand{\range}{\mathrm{range}\,}\)
\( \newcommand{\RealPart}{\mathrm{Re}}\) \( \newcommand{\ImaginaryPart}{\mathrm{Im}}\)
\( \newcommand{\Argument}{\mathrm{Arg}}\) \( \newcommand{\norm}[1]{\| #1 \|}\)
\( \newcommand{\inner}[2]{\langle #1, #2 \rangle}\)
\( \newcommand{\Span}{\mathrm{span}}\)
\( \newcommand{\id}{\mathrm{id}}\)
\( \newcommand{\Span}{\mathrm{span}}\)
\( \newcommand{\kernel}{\mathrm{null}\,}\)
\( \newcommand{\range}{\mathrm{range}\,}\)
\( \newcommand{\RealPart}{\mathrm{Re}}\)
\( \newcommand{\ImaginaryPart}{\mathrm{Im}}\)
\( \newcommand{\Argument}{\mathrm{Arg}}\)
\( \newcommand{\norm}[1]{\| #1 \|}\)
\( \newcommand{\inner}[2]{\langle #1, #2 \rangle}\)
\( \newcommand{\Span}{\mathrm{span}}\) \( \newcommand{\AA}{\unicode[.8,0]{x212B}}\)
\( \newcommand{\vectorA}[1]{\vec{#1}} % arrow\)
\( \newcommand{\vectorAt}[1]{\vec{\text{#1}}} % arrow\)
\( \newcommand{\vectorB}[1]{\overset { \scriptstyle \rightharpoonup} {\mathbf{#1}} } \)
\( \newcommand{\vectorC}[1]{\textbf{#1}} \)
\( \newcommand{\vectorD}[1]{\overrightarrow{#1}} \)
\( \newcommand{\vectorDt}[1]{\overrightarrow{\text{#1}}} \)
\( \newcommand{\vectE}[1]{\overset{-\!-\!\rightharpoonup}{\vphantom{a}\smash{\mathbf {#1}}}} \)
\( \newcommand{\vecs}[1]{\overset { \scriptstyle \rightharpoonup} {\mathbf{#1}} } \)
\( \newcommand{\vecd}[1]{\overset{-\!-\!\rightharpoonup}{\vphantom{a}\smash {#1}}} \)
\(\newcommand{\avec}{\mathbf a}\) \(\newcommand{\bvec}{\mathbf b}\) \(\newcommand{\cvec}{\mathbf c}\) \(\newcommand{\dvec}{\mathbf d}\) \(\newcommand{\dtil}{\widetilde{\mathbf d}}\) \(\newcommand{\evec}{\mathbf e}\) \(\newcommand{\fvec}{\mathbf f}\) \(\newcommand{\nvec}{\mathbf n}\) \(\newcommand{\pvec}{\mathbf p}\) \(\newcommand{\qvec}{\mathbf q}\) \(\newcommand{\svec}{\mathbf s}\) \(\newcommand{\tvec}{\mathbf t}\) \(\newcommand{\uvec}{\mathbf u}\) \(\newcommand{\vvec}{\mathbf v}\) \(\newcommand{\wvec}{\mathbf w}\) \(\newcommand{\xvec}{\mathbf x}\) \(\newcommand{\yvec}{\mathbf y}\) \(\newcommand{\zvec}{\mathbf z}\) \(\newcommand{\rvec}{\mathbf r}\) \(\newcommand{\mvec}{\mathbf m}\) \(\newcommand{\zerovec}{\mathbf 0}\) \(\newcommand{\onevec}{\mathbf 1}\) \(\newcommand{\real}{\mathbb R}\) \(\newcommand{\twovec}[2]{\left[\begin{array}{r}#1 \\ #2 \end{array}\right]}\) \(\newcommand{\ctwovec}[2]{\left[\begin{array}{c}#1 \\ #2 \end{array}\right]}\) \(\newcommand{\threevec}[3]{\left[\begin{array}{r}#1 \\ #2 \\ #3 \end{array}\right]}\) \(\newcommand{\cthreevec}[3]{\left[\begin{array}{c}#1 \\ #2 \\ #3 \end{array}\right]}\) \(\newcommand{\fourvec}[4]{\left[\begin{array}{r}#1 \\ #2 \\ #3 \\ #4 \end{array}\right]}\) \(\newcommand{\cfourvec}[4]{\left[\begin{array}{c}#1 \\ #2 \\ #3 \\ #4 \end{array}\right]}\) \(\newcommand{\fivevec}[5]{\left[\begin{array}{r}#1 \\ #2 \\ #3 \\ #4 \\ #5 \\ \end{array}\right]}\) \(\newcommand{\cfivevec}[5]{\left[\begin{array}{c}#1 \\ #2 \\ #3 \\ #4 \\ #5 \\ \end{array}\right]}\) \(\newcommand{\mattwo}[4]{\left[\begin{array}{rr}#1 \amp #2 \\ #3 \amp #4 \\ \end{array}\right]}\) \(\newcommand{\laspan}[1]{\text{Span}\{#1\}}\) \(\newcommand{\bcal}{\cal B}\) \(\newcommand{\ccal}{\cal C}\) \(\newcommand{\scal}{\cal S}\) \(\newcommand{\wcal}{\cal W}\) \(\newcommand{\ecal}{\cal E}\) \(\newcommand{\coords}[2]{\left\{#1\right\}_{#2}}\) \(\newcommand{\gray}[1]{\color{gray}{#1}}\) \(\newcommand{\lgray}[1]{\color{lightgray}{#1}}\) \(\newcommand{\rank}{\operatorname{rank}}\) \(\newcommand{\row}{\text{Row}}\) \(\newcommand{\col}{\text{Col}}\) \(\renewcommand{\row}{\text{Row}}\) \(\newcommand{\nul}{\text{Nul}}\) \(\newcommand{\var}{\text{Var}}\) \(\newcommand{\corr}{\text{corr}}\) \(\newcommand{\len}[1]{\left|#1\right|}\) \(\newcommand{\bbar}{\overline{\bvec}}\) \(\newcommand{\bhat}{\widehat{\bvec}}\) \(\newcommand{\bperp}{\bvec^\perp}\) \(\newcommand{\xhat}{\widehat{\xvec}}\) \(\newcommand{\vhat}{\widehat{\vvec}}\) \(\newcommand{\uhat}{\widehat{\uvec}}\) \(\newcommand{\what}{\widehat{\wvec}}\) \(\newcommand{\Sighat}{\widehat{\Sigma}}\) \(\newcommand{\lt}{<}\) \(\newcommand{\gt}{>}\) \(\newcommand{\amp}{&}\) \(\definecolor{fillinmathshade}{gray}{0.9}\)Of all thermal piston engine types, the diesel engine has the best thermo- dynamic efficiency – significantly higher than that of gasoline engine, and overwhelmingly higher than that of steam engine. The economy of diesel cars, trucks, locomotives, bulldozers and ships is therefore much better than that of their counterparts powered by other engine types. The drivers of diesel cars complained about their “sluggishness”, i.e., their poor acceleration. Therefore, over the recent decades much effort has been devoted to “improving the muscle” of diesel engines for passenger cars. New lightweight turbocharged diesel engines with sophisticated combustion chambers gave the cars a vigor similar to that of gasoline cars. However, it badly worsened the emission of noxious pollutant in the exhaust. Therefore, some European countries are seriously considering closing city centers for diesel cars. Obviously, it would mean a dramatic drop of interest in diesel cars from customers. Not surprisingly, some major manufacturers have already announced that they will discontinue the production of diesel cars.
But in the area of vehicles which are “workhorses” the death of diesel engines doesn’t seem to be so imminent. The engines for buses, trucks, tractors and locomotives don’t need engines giving them a temper of racing cars. In the case of engines that are only supposed to be “workhorses” it is much easier to design such that have lower emission than “muscle” diesels, and it is also easier to develop efficient exhaust filters for them. And there is one more reason why “workhorse” vehicles with diesel engines will not disappear soon from the roads, railway tracks, and construction sites: there is simply no alternative propulsion for them. Small passenger cars with diesel and gasoline engines may be replaced by zero-emission cars (electric or fueled by hydrogen) or low emission cars (such as plug-in hybrids), but there is no viable alternative of such kind for tractor-trailers making nearly one thousand miles per day.
So, if there are no prospects from eliminating diesel vehicles from the road in foreseeable future, what can we do? Well, we can make fuels for such vehicles that will make them as “environmentally friendly” as possible.
Petroleum Diesel Fuel
Natural petroleum crude oil is a mixture of an incredible variety of hydro- carbons, the approximate chemical formula of which can be given as CnH2n. The overwhelming majority of the molecules have n between 5 and 40. In refineries crude oil is separated into fractions, the lighter of which is gasoline, with the average value of n = 8. Diesel fuels is a mixture of molecules with carbon numbers between n = 8 and n = 24, with the average carbon number
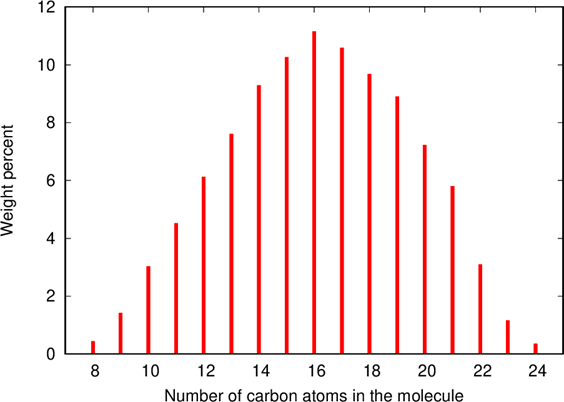
Kerosene is pretty similar to diesel fuel, it’s a mixture of molecules with carbon number \( n \) between 10 and 16. Kerosene is essentially the same as the aviators call jet fuel.
The hydrocarbon molecules in diesel fuel and in kerosene have a large variety of shapes and structures. Some of them are aromatic hydrocarbons containing rings made up of 6 carbon atoms. Some are linear chains with or without sub-chains branching out.
Fatty Acids and Fats
Fatty acids are important biomolecules – they do not occur alone plant or animal tissues, but they are essential “building blocs” for a class of substances known as lipids. Lipids play important roles in living organisms, both in biochemical processes and as structural components of tissues. For instance, cell membranes in animal (and human) tissues are made of phospholipids. Cholesterol – everybody has heard of it – is also a lipid. Our brains are made in a large part of lipids. And yet another important sub-class of the lipid family are fats, used both by animals and by plants as energy storing substances. In this section, we will limit our attention to fats only.
The most common fatty acid occurring in animal and plant fats is the palmitic acid. Its condensed chemical formula is C16H32O2. It consists of a linear hydrocarbon chain with 15 carbon atoms, CH3(CH2)14– , which constitutes a so-called alkyl group2 to which there is attached a so-called carboxyl group –(CO)OH, which occurs in a great many of organic acids, including the well-known acetic acid (vinegar) and citric acid. The structural formula of palmitic acid is shown in Fig. 10.13:
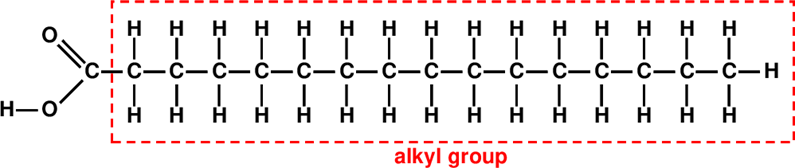

In organic chemistry, if we attach a carboxylic group –(CO)OH to any alkyl group, we obtain an acid. But if we attach a hydroxyl group –OH in- stead, we obtain an alcohol. For instance, the simplest of all alkyl group (and a very important one!) is the methyl group CH3– . By attaching a hy- droxyl group –OH one obtains CH3–OH, methyl alcohol, or methanol. Ethyl, CH3CH2– plus -OH yields ethyl alcohol, or ethanol, our good friend (but not too good, I hope!). And propyl CH3CH2CH2– plus –OH yields propanol 2.

There may be more -OH groups than a single one – for instance, if one replaces two more hydrogen atoms in propanol by hydroxyl groups, the result is glycerol, commonly known as glycerin. It is a viscous, odorless liquid, non- toxic and tasting sweet.
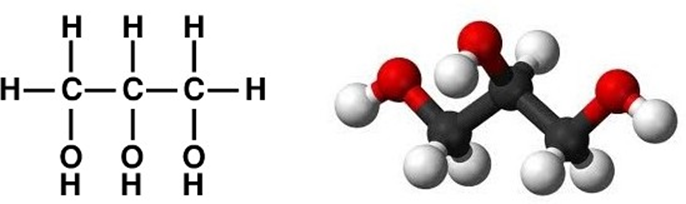
Now, the only thing that needs to be done before we can explain how fats are built, is to explain what the esterification reaction is. It is in certain measure analogous to the acid-base reaction in inorganic chemistry, which produce a salt molecule plus a water molecule, for instance: NaOH + HNO3 NaNO3 + H2O. The two reagents in esterification are an alcohol and an organic acid. For example, the esterification reaction between methanol and acetic acid, or – according to the most recent rules in organic chemistry – ethanoic acid, yields an ester called methyl ethanoate plus a water molecule.

An ester is therefore an analog of a salt in inorganic chemistry.
OK, now we can finally tell what the fats are: they are esters called triglyc- erides – each molecule is derived from a single glycerol molecule and three fatty acid molecules.
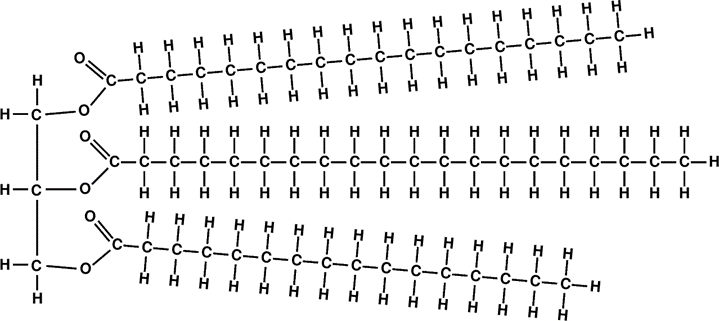
It should be noted that there is a great variety of naturally occurring fatty acids. All those we have discussed so far are saturated fatty acid, meaning that there are two hydrogen atoms attached to each carbon atom in the alkyl group, with the exception of the last carbon, which has three hydrogen atoms. In natural fatty acids the number of carbon atoms are always even.
There are ten saturated acids with the number of carbon atoms from 8 to 26.
But the saturated acids are a small minority in the fatty acid family. The thing is that in the hydrocarbon tails there may be unsaturated bonds – it means that a pair of carbon atoms is connected not by a single, but by a double bond – and consequently, there is only a single hydrogen atom connected to each carbon atom. Fatty acids with a single bond of such kind are simply called unsaturated. But there may be two such bonds in the chain, then the acid is doubly unsaturated. And the unsaturated bonds may be at different positions. And last, but not least, the unsaturated bonds may be of two types, trans or cis. So, the bad news is that there are “zillions” of possible combinations of triglycerides.
The bad news is that all such combinations are not equivalent. At least, depending on the circumstances. If a fat is intended for human consumption, the properties of constituent fatty acids may really matter. American Heart Association recommends limiting consumption of saturated fats, found primarily in animal products. Also, trans- fats should be avoided. In short, the terms trans and cis apply to the properties of unsaturated bonds in fatty acids. A cis-type double bond produces a characteristic “kink” in the fatty acid hydrocarbon chain – while a chain with a trans-type double bond remains straight. Unsaturated plant fats contain almost exclusively cis- type fatty acids. Trans fats are present in animal fats, most of all in dairy product, and the highest content may be found in the so-called “artificially hydrogenated oils”, e.g., in margarines and in shortenings3.
But the good news is that if fat is to be used as a feedstock for making biodiesel, then it really doesn’t matter whether the fatty acids in it are satu- rated or unsaturated and of the cis-or the trans-type. Even non-edible plant fats can be converted to biodiesel.
Converting Fats to Biodiesel: Transesterification
Essentially, conversion is not absolutely necessary. In one of the first pub- lic demonstrations of his invention, at the 1900 World Fair in Paris, Rudolf Diesel used peanut oil as the engine’s fuel. Likewise, some biodiesel ama- teurs fuel their cars with cooking oil (often spent frying oil they buy from fast-food restaurants). It requires some modification of the engine’s fuel sys- tem because cooking oil is far more viscous that regular diesel fuel – the car owners solve this problem by installing an extra electric heater in the fuel line that heats up the oil to about 180◦ F, at which temperature its viscosity is approximately the same as that of petroleum diesel fuel. However, this is considered an unsophisticated and not very elegant method by more ambi- tious biodiesel enthusiast, the more that such converted automobile has to run on cooking oil only, one cannot mix it with regular diesel fuels.
But for those ambitious biodiesel enthusiast, if they have a source of vegetable oil – there is an “elegant” option: namely, one can use a process of transesterification. It’s relatively simple and can be done even in one’s garage using a relatively uncomplicated apparatus.
So, what is the transesterification reaction? Suppose that there is an organic acid \(\Phi-C O O H\) and an alcohol HO\(\Omega\) - where \(\Phi\) and \(\Omega\) are alkyl groups (let's recall: an alkyl group is a saturated hydrocarbon minus one hydrogen atom). Now, let them form an ester:
\[ \Phi-\mathrm{COOH}+\mathrm{HO}-\Omega \longrightarrow \Phi-\mathrm{COO}-\Omega+\mathrm{H}_{2} \mathrm{O} \]
Like in Fig. \(\PageIndex{6}\), right? And now, suppose, another alcohol HO- \(\Delta\) enters the stage, and something like a "love affair" happens: the acid brakes the relationship (the ester link) with \(\Omega\), "dumps" it, and instead form an "ester relationship" with the HO- \(\Delta\) alcohol:
\[ \Phi-\mathrm{COO}-\Omega+\mathrm{HO}-\Delta \longrightarrow \Phi-\mathrm{COO}-\Delta+\mathrm{OH}-\Omega \]
In other words, the acid de-esterifies itself from the first alcohol, and then it re-esterifies itself with the other alcohol. This is how transesterification works.
The triglycerides are triple esters that forms when three fatty acid molecules combine with a single glycerol molecule which is a triple alcohol. But it turns out that fatty acids have a stronger tendency to “get paired” with some single alcohols than with glycerol. For instance, with methanol, ethanol, or propanol (see Fig. \(\PageIndex{4}\)).
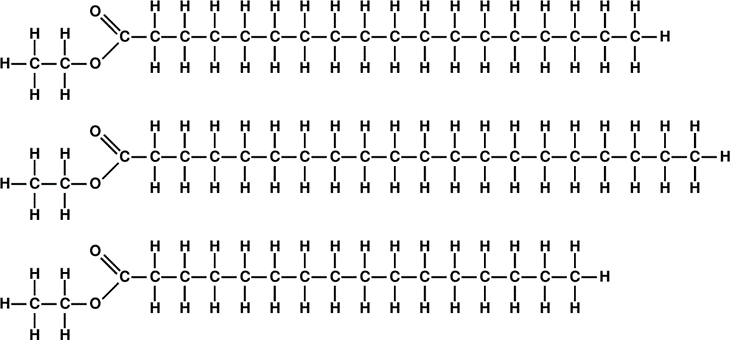
What is the sense of performing transesterification? From Figs. \(\PageIndex{7}\) and \(\PageIndex{8}\) one can find, after doing some tedious, but straightforward arithmetic, that the chemical formula of the triglyceride molecule is C57H110O6, and its molecular mass is 57 × 12 + 110 × 1 + 6 × 16 = 890. And the average formula for the three ethyl esters is C20H40O2, with the average molecular mass of 312.
The high molecular mass of constituent molecules makes a fluid viscous. By performing transesterification, one obtains a mixture of esters with an average composition quite similar to that of petroleum diesel fuel – with only two extra oxygen atoms per molecule, which in the case of molecules that large makes only a marginal difference.
Practical Methods of Transesterification
As noted, there are transesterification recipies that can be carried out in one’s garage or in kitchen. There is one more detail that has not been mentioned in the preceding section: namely, it’s not enough to mix oil with alcohol. There has to be a catalyst in the mixture – either inorganic or organic. In home production of biodiesel it is easier to use an inorganic catalyst – its role is to provide a strong enough alkaline environment. NaOH, KOH or even quicklime may be used – the only disadvantage is that a not-so-small amount has to be used. As far as alcohol is concerned, one can use methanol, ethanol or propanol. Ethanol option, if one has no chance of purchasing industrial stuff and goes instead to a liquor store, may be pretty expensive. Industrial methanol is sold by hardware stores – but it is highly toxic. A much better choice may be isopropyl alcohol, widely used as a disinfectant – it’s inexpensive and readily available in pharmacies and grocery stores.
It would not make sense to provide a detailed recipe for home-making biodiesel in this text, because a whole bunch of really instructive ones is available at YouTube. The Author has reviewed quite a few of them and can give some recommendations:
A non-nonsense 14m30s movie, the author does not speak a single word, but everything is made clear by how things are shown. A nice thing is that at the end it is shown that the biodiesel really works!
https://www.youtube.com/watch?v=vK8HmWurJow no longer there
University of Idaho tutorial, 5m53s, a nice mixture of theory and practice. KOH and ethanol.
• Omaha Biodiesel Coop, 9m10s, NaOH (lye) and methanol recipe.
Industrial enterprises use more sophisticated technologies – one difference compared with the simple procedures one can carry out in one’s garage is us- ing an enzymatic catalyst. Here is a short video published by TransBiodiesel Ltd. It’s impressive how little enzyme catalyst is needed – only 1 weight unit per 3000 - 4000 weight units of vegetable oil.
Energy Balance and Global Production of Biodiesel
Industrial companies use oils from many different sources: e.g. canola oil, soybeans oil, or palm oil. The energy balance one can find in literature varies depending on the source. One study finds that for small farms it may be close to 2. The figure given by Wikipedia is 2.6. It’s definitely better that 1.3 for corn ethanol, but still far from the value of 8.3 for Brazilian sugar cane ethanol. But it seems that there is still room for optimism, because in one study published in 2011 a record-high value of 5.5 has been reported.
In Table 1 typical yields of biodiesel per acre are shown for several different crops (more data can be found in this Wikipedia article. There is one clear conclusion emerging from the yield data – namely, as with the bioethanol production, the tropical regions are “privileged”.
Table 1. Typical yield of biodiesel per acre
Crop |
Gallons per acre |
---|---|
Palm oil |
508 |
Coconut |
230 |
Rapeseed (canola) |
102 |
Soybeans |
59-99 |
The global biodiesel production by feedstock data for the years 2007-19 are shown in Fig. 10.20. The current production is approxing 40 biilion liters per year, which is about 1.5% of the US petroleum diesel consumbtion per year and about 0.5% of the global consumption of petroleum diesel fuel. From Fig.
10.20 it follows that it took about 9 years for the global biodiesel production to double. So, assuming that the demand for diesel fuel remains unchanged over the years to come, with the current biodiesel production growth rate it may take about 60 years for the biodiesel production to satisfy 50% of the total demand for diesel fuel. Not a very optimistic scenario, right? But 60 years is a long time horizon. Many things may happen. Global petroleum diesel fuel production has doubled since 1988, so our assumption that it will stay unchanged from now on is a bit risky. On the other hand, global resources of oil will not last forever. New and new petroleum oil deposits are being discovered, but it is hard to make trustworthy predictions reaching more than half a century ahead.
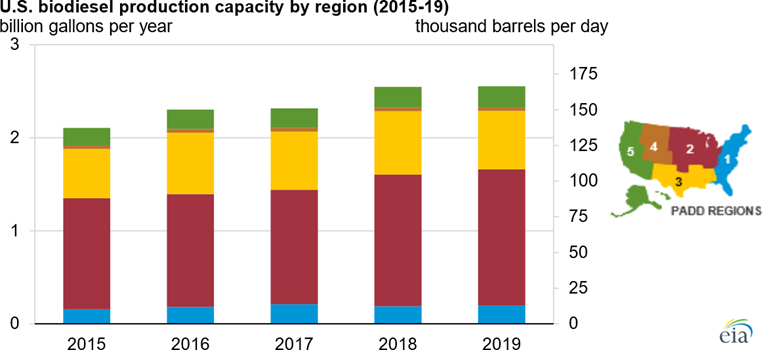
Algae Diesel Fuel
Currently, about 75% of word biodiesel is derived from vegetable oils – extracted from oil palm fruits, coconuts, rapeseed, soybeans, sunflower seeds, and a few other seeds of oily-rich species. All those plants have been cultivated for centuries for human consumption. When the biodiesel industry emerged, it had an “easy start”, because much of the needed infrastructure and “know-how” had already been created: how to cultivate the plants, how to harvest the oily parts and how to extract the oil. What remained to be done was to develop the technology of esterification – and, as follows from the preceding sections, it’s a relatively straightforward technological process. All the above-listed plants are terrestrial-based. There are, however, plants living in water environment, which are far more efficient lipid producers than the oil palm, the record-keeping terrestrial-based plant. They are members of the algae family, an extra-originally large one with about 100 000 different fresh-water and sea-water species. Some algae, if farmed, may yield a crop per acre as much as 50 or even more times larger than the crop from the best land-based oily plants. The US Department of Energy estimates that algae fuel from farms occupying only 0.42% of the US territory would be able to provide enough fuel for replacing all the petroleum-based fuels currently used in the US. And it’s only 1/7 of the land area currently used for corn growing!
If so, why don’t we shut down all the farms growing feedstock for corn ethanol and vegetable biodiesel and replace them with algae growing farms? Well, the problem is that the algae biofuel industry is currently at its “earlest infancy”. Vegetable oil plants have been harvested for centuries (or even for several thousand years, as is the case with olive trees). But until quite recently, nobody was trying to grow and process algae at an industrial scale. Currently, there is lab research and there are some small-scale pilot research projects going on. However, it is beyond doubt that creating a sustainable algae biodiesel industry will require creating an enormous infrastructure. But almost nothing has been built so far. Even worse, at the moment it’s not even clear what should the new industry look like: fields of open ponds, or closed bio-reactors in giant greenhouses? Plus a whole bunch of other ques- tions. There are many visions and concepts, some of them in conflict with other visions and concepts. Among experts, there are optimistic and even enthusiastic voices. Here are some samples: one from Exxon, another one also from Exxon, one from the Department of Energy’s Bioenergy Technolo- gies Office. Here is one much more sceptic voice, one clearly pessimistic, and another one expressing similar doubts whether sustainable algal biofuel production is at all possible? Well, even if it is, the “time horizon” given in the “optimistic” Department of Energy Bioenergy Technologies Office’s document linked above is not per se very optimistic. Please look at Page 27 of the said document, where the goals are stated: five billion gallons per year of algal biofuel by the year 2030. Well, today’s (2018) biodiesel production according to the US EIA is 1.8 billion gallons, and the total consumption of petroleum diesel fuel is slightly over 60 billion gallons. As follows from these numbers, According to the most ambitious goals, the algal biodiesel production will not even reach 10% of the diesel fuel consumption in 2030. So, we have to be realists! We can start singing “It’s a long way to get rid of petroleum diesel”, to the tune of It’s a long way to Tipperary, a famous World War I song. But eventually this goal must be reached!
____________________________________________________________
1. An alkyl group is alkane minus one hydrogen atom – and alkane, a.k.a. paraffin, is any of the series of saturated hydrocarbons CnHn+2.
2. The physical properties end the smells of all these three alcohols are very similar – and methanol, if consumed, may cause blindness, or even death.
3. Our ancestors living at tree-tops for millions of years fed mostly on plant products, fruits and nuts, which contained almost exclusively cis fats. Meat appeared in their diet much later, and diary products with high concentration of trans fats only tens of thousands years ago. Perhaps Mother Evolution did not have enough time to teach our organisms to tolerate the trans fats, and this is the reason why we should – well, not to avoid trans fats completely, but at least show moderation in consuming them.