Chapter 2: Polymerization
( \newcommand{\kernel}{\mathrm{null}\,}\)
Just a reminder from last time that polymers are composed of monomers, or structural units, and these monomers are repeated approximately 100 times for oligomers and more typically 10-100k for typical macromolecules/polymers and we had the following reaction for polyethylene which utilized ethylene as a monomer, or structural unit, and also has ethylene as the repeat unit.
Remember that n is the just the number of time we repeat the repeat unit, not trying to repeat myself. We talked last time as well about some of the parameters of polymer chains that we are interested in some of those being chain architecture, isomer states, etc. But one of the key parameters that we also care about is the molecular weight/molar mass and the molecular weight/molar mass distribution (units in). To be more accurate it is better to use the term molar mass but you will typically see the term molecular weight in other literature quite often. These values are critical as at high molar masses the viscosity increases dramatically as does the ultimate tensile strength typically.

Now let’s first talk about how we calculate the molar mass. For a single homopolymer (composed of only one polymer unlike co-polymers or block-copolymers), like that for polyethylene above, would simply be:
M=nMr
where M is the molar mass of the homopolymer, n is the number of times the repeat unit is repeated, and Mr is the molar mass of the repeat unit. However, typically when we synthesize polymers not all polymers will be of exactly the same length as it is a chemical reaction which polymerizes the monomers.
Thus we are typically concerned with average molar mass values, M. And there are two primary ways we will calculate the average molar mass, those being the number-average, Mn, and weight-average, Mw, molar mass.
The number-average molar mass is sum of the products of the molar mass of each fraction multiplied by its mole fraction
¯Mn=∑XiMi
where Xi is the mole fraction corresponding the the molar mass Mi. This can also be written as
¯Mn=∑NiMi∑Ni
where Ni is the number of polymers with molar mass Mi. You’ll notice that this is simply the ratio of the total mass divided by the number of chains.
The weigh-average molar mass is the sum of the products of the molar mass of each fraction multiplied by it’s weight fraction or
¯Mw=∑wiMi
where wi is the weight fraction. The weight fraction is simply the mass of molecules with molar mass mi divided by the total mass
wi=NiMi∑NiMi
so we can re-write the weight average molar mass as
¯Mw=∑NiM2i∑NiMi
An example of a typical molar mass distribution for polymers can be seen here. Notice that the weight averaged is larger than the umber average. This must be the case as the larger polymers weight more the weight average is always skewed to the right of the number average.
We can now define another very useful quantity called the polydispersity index or PDI. This is essentially a measure of the breadth of the molar mass distribution and it is
PDI=¯MwMn
This value typically ranges from 1.5-2.0 for many polymers but can range from 1.05-50. Note that for a perfectly monodisperse polymer the PDI values would be 1. We can never be less than 1!
Let’s do a quick problem
Ex. 1:

Calculate the Mn,Mw, and PDI of a polymer sample created by mixing 1 mole of a 50,000 g/mol polymer with 1 mole of a 150,000 g/mol polymer chain.
Solution
Add example text here.
Finally the last parameter that we have neglected to discuss so far when characterizing the polymerization process is the one that accounts for the chemical nature of polymerization and that is the the number-average, Dp,n and weight-average Dp,w degree of polymerization. These values are simply defined as
¯Dp,n=¯MnMr
¯Dp,w=¯MwMr
notice in the above expressions that these values are a function of the the number or weight of the polymers as expected but they are also functions of p which is a new parameter the extent of the reaction/conversion. This quantifies how the extent of the chemical reaction and conversion of monomers to polymers. This value will depend on many factors (temperature, pH, etc.). We will talk more about this and how to relate it to reaction kinetics in just a minute.
Let’s do another quick problem
I have a sample of polyethylene oxide (PEO) where all the chains have number average degree of polymerization of 10,000 and is degraded chemically into equal molar amounts of chains with a Dp,n of 7000, 2000, and 500. What is the Mn,Mw, and PDI of the polymer sample before and after degradation.
Solution
Add example text here.
So we now have the tools to characterize our polymer sample after it has been polymerized but how do we go about actually polymerize our polymer? Well I hope everyone is ready for a tiny bit of organic chemistry!! Yeah!
Polymer Chemistry/Polymerization
As we have discussed previously polymerization in its most basic form is linking at least two monomers together via a chemical reaction. So you can imagine there are too many different types of polymerization reactions to cover in this course so we will only highlight a few but I can give you resources to investigate further if you are so inclined to do so. I would also suggest taking an organic chemistry course or two as well if you are interested in polymer synthesis. We can generally classify polymerization into two broad categories distinguished by the underlying polymerization mechanisms. Those two categories are step-growth and chain-growth polymerization. In step-growth polymerization the polymer chains grow by step-wise reactions whereas in chain-growth polymerization requires an initial reaction between the monomer and an initiator to start the growth of the chain. This is also expressed below as
Chain Growth PolymerizationMn+M→Mn+1
Step Growth PolymerizationMx+My→Mx+y+HCl,H2O
Where for chain growth you simply add (sometimes referred to as addition polymerization) a monomer of the same type to grow a polymer chain. For step-growth polymerization you typically utilize two different monomers which will react chemically and produce a new polymer with 2 structural units and 1 repeat unit and there is typically some by product from the reaction typically water or some acid. Additionally the monomer will be present till the end of the reaction for chain growth while the monomer will disappear at very early stages of the reaction. Finally the mechanism by which chain growth occurs is via three steps
- Initiation
- Propagation
- Termination
The step growth mechanism occurs via independent reactions of functional groups at chain ends. We will talk about this more in depth right now!


Step-Growth Polymerization
For step-growth polymerization or simply step polymerization the key characteristics of this type of polymerization is that the monomer will typically disappear at the very early stages of the reaction. As the extent of the reaction progress, p, the molecular weight is initially very low but at the end of the reaction is increases dramatically. Finally, the mechanism of this type of polymerization is via independent reactions of functional groups at chain ends. Additionally, in order to have efficient step polymerization, i.e. high molecular weight, we need to have
- Exact equivalence of functional groups
- Very high degree of conversion
- No side reactions
- No monofunctional impurities
Some step growth polymers include esters, amides, carbonates, urethanes, and ureas as seen here as well as some functional groups.

So if we want a high molecular weight how can we achieve this? Well via extent of the reaction and the reactant ratio which we will discuss right now.
Controlling Molar Mass Via Extent of Reaction and Reactant Ratio: The Carothers Equation

We can define the extent of the reaction or conversion p as simply the ratio of the number of functional groups remaining over the number initially present
p=1−NNo
where N is the number of molecules at some time ,t and No is the number of molecules initially present. You can also break it down further by the extent to the reaction of functional groups let’s say we are trying to polymerize RA2 and RB2, seen here,

then we can write the extent of the reaction for both functional groups
pA=1−NANA,o
pB=1−NBNB,o
where NA,o is the number of initial functional groups A and the same for B.
You will notice that we can now re-write this as
p=1−1¯Dp,n
As we have hinted at previously in order to achieve the largest molar mass possible we need to have exact stoichiometric ratios and we can quantify this using the reactant ratio r which is simply
No=NA+NB2
Note we divide by 2 because there are two functional groups per molecule. And we can re-write this equation as follows
No=NB(1+r)2
So we again are working toward an equation that will relate how the number averaged molar mass is controlled by the extent of the reaction and the reactant ratio. Well after some time how many unreacted A and B groups will there be? It should be
NA−pNA
NB−pNA
Notice that because we have previously indicated that A is in the minority the reaction will obviously stop once we hit our limit of A so we have indicated that above. We can also write the expression above equivalently in terms of the majority component B
rNB(1−p)
NB−prNB
So now we can express the number of molecules remaining at some time t which is
N=NB(1+r−2rp)2
So now we can write an expression for how the \textbf{number averaged molar mass is controlled by the extent of the reaction and the reactant ratio}
¯Dp,n=NoN=1+r1+r−2pr
This is the most general Carothers equation and can also be arrived at using a probabilistic perspective and if you are interested in this you can borrow by Young and Lovell text if you are interested in the derivation. Let’s stop one quick moment and appreciate how powerful this equation is. By simply changing the reactant ratio or by waiting for the reaction to proceed via the rate of the reaction we can control the molar mass!! You can see the effects in the Mathematica Demonstration.
Reaction Kinetics
We have talked a lot about the extent of the reaction and that it will change as a function of time. So what are the kinetics that control this process? Well let’s answer that by returning to our old friends, the equilibrium constants. So let’s start by taking a look at a very general and generic step polymerization reaction.
where you can see that the A and B functional groups react to form a new linkage between the two monomers (red and blue), C, and there is an additional byproduct, D.

For this reaction we know that the equilibrium constant Keq is
Keq=kfkr=[C][D][A][B]
where kf is the forward reaction rate constant, kr is the backward reaction rate constant, and [ ] denotes concentrations of each respective component. Also it should be noted that at equilibrium the rate of forward reaction, Rf, is equal to the rate of the reverse reaction Rr. And we have that
Rf≈kf[A][B]
Rr≈kr[C][D]
Now for polymerization we want the rate constant to be very high, 104, because this indicates that we are creating more products than reactants which is great because we want to end up with our polymer. If the Keq≈1 then we will have to do something externally to bias the reaction forward like removing some of the byproducts of the reaction, i.e. Le Chatelier′s principle. Again this is great but how does this polymerization actually occur.
Nucleophilic Acyl Substitution
One of the most common chemistries/methods of linking monomers together is via nucelophilic acyl substitution. A nucelophile is an electron rich species that will react with an electron poor species by donating an electron. A nucleophile seeks out electron poor sites. An electrophile an electron poor species that will react an electron rich species by accepting a pair of electrons. An electrophile will seek out electron rich sites. So the nucleophile will attack the electrophile, form an intermediate, and then leaving group will depart. Here you can see how the nucleophile attacks the electron poor carbon and then kicks out the leaving group LG.

Or even more generally for a polymer

Let’s look at the acyl group. Which is the nucelophile and which is the electrophile? Well O is the nucelophile and C is the electrophile as the C=0 bond is polar and O grabs the electrons, because it is electronegative.
For most of the carboxylic acids that we will deal with in the following polymerization methods it is important to remember the relativity reactivity of the groups which is as follows, with approximate Keq.
Direct, Exchange, and Acid Chloride Polymerization Methods
With that background let’s make our first polymer!!! Some of you are probably wearing it right now, polyester. We will make polyester using three mechanisms

- Direct Esterification
- Ester Exchange with Alcohols
- Reactions of Acid Chlorides
Let’s start with direct esterification and remember this we are still talking about step-growth polymerization.
Direct Esterification
Here we can see a general direct esterification reaction and we can see that the byproduct is water. Therefore, this is considered a condensation reaction which is a reaction where small molecules are lost as byproducts when forming the larger polymer chain. Now this is an essential point to make here and why we went to the trouble of talk about the different equilibrium constants. If this reaction has a rate constant Keq ≈ 1 we either need to increase temperature drastically or remove the water to bias the reaction forward. For this reaction we may need to remove water and of course we need to have equal stochiometry.
Ester Exchange with Alcohols
For this reaction we also may need to remove alcohol due to the reaction equilibrium constants and we need to have equal stochiometry.
Reactions of Acid Chlorides


For this reaction luckily we don’t have to remove HCL because our reaction equilibrium is much higher as we remember from the table that we introduced earlier.
However we will again have to have equal stochiometry and as the reaction proceeds if the solution becomes too acidic you might have to add a base to the solution.

Making Polymers! Finally!
Now that we have seen several general reactions let’s start actually making some polymers. Let’s start by making polyehtylene adipate from adipic acid and ehtylene glycol using direct esterification.
How about making polyethylene terephalate (PET) from terephalic acid and ethylene glycol.
Let’s make this polymer another way using ester exchange
Note that in this reaction there are two steps first is our typical exchange and we have to remove the byproduct. By continuing to heat the sample our products from the first step will react with one another producing polythylene which will react with the terephalic acid from the first step.
Now let’s make some polycarbonates (PC). We can do so via reactions with phosgene or via our typical interchange reaction
Let’s make some polyamides as well and we can do so via direct amidation. One quick note here is that the reaction that creates the amide linkage can have a Keq ≈100 − 1000 so we may not necessarily have to remove the water in this reaction, as we saw with our Nylon 6,6 demonstration.




or via reactions with diacid chlorides.


Also take a moment to appreciate the implication of these amide linkages. In a polymer chain these amide linkages can form hydrogen bonds with other polymer


chains or even with itself and this will have vast implication for polyamide mechanical and dynamical properties.
Now let’s make some aromatic polyamides, specifically Kevlar which has the ultimate tensile strength of steel at of the weight! When making aromatic polyamides you have to use an acid chloride because the aromatic ring with the NH2 exhibits resonance electron delocalization so it is a weak nucleophile so we need to have the most reactive group for the reaction to proceed. Also typically to increase the yield of this reaction it will typically take place in a solution with catalyzing agents like DMF and LiCl, or even H2SO4 solutions.

Finally let’s talk about synthesizing a very important polymer, polypeptides/proteins (although proteins will typically have a 3D structure and contains many many peptides). Here we show a general reaction with an R group but it can be any of the 21 amino acids shown in the table here.
In general though you can imagine that if we want to make sure that the reaction proceeds without interacting with these R groups we will most likely have to take steps to protect these R groups. That amount of chemistry is beyond the scope of this class but I can point you to resources if you are interested.
With this we have exhaustively talked about a number of polymerization methods but remember there are many other methods and if you are interested there is some literature or external sources of information that might help you find the appropriate mechanism for your material of interest.
Chain-Growth Polymerization:
For chain-growth polymerization or more simply chain polymerization monomer units are attached one unit at a time to an ever elongating polymer chain. Chain polymerization can contain the following steps
(1) Initiation
(2) Propagation
(3) Chain Transfer - Chain is terminated but the active site is transferred to a new chain and typically results in more branching. This step doesn’t always occur.
(4) Termination
Additionally, the monomer is present until the end of the reaction. Also unlike step polymerization, the molecular weight for chain polymerization initially shoots up and then asymptotically increases very slowly as the extent of the reaction, p, increases. Some chain growth polymers include PVC, PS, and polypropylene (PP).

Figure 26. Chain vs. Step Growth.
Some common chain growth monomers are vinyl groups, ketones, aldehydes, and heterocyclic ring monomers (strained). And some common propagating or active species include anionic, cationic, and free radicals.
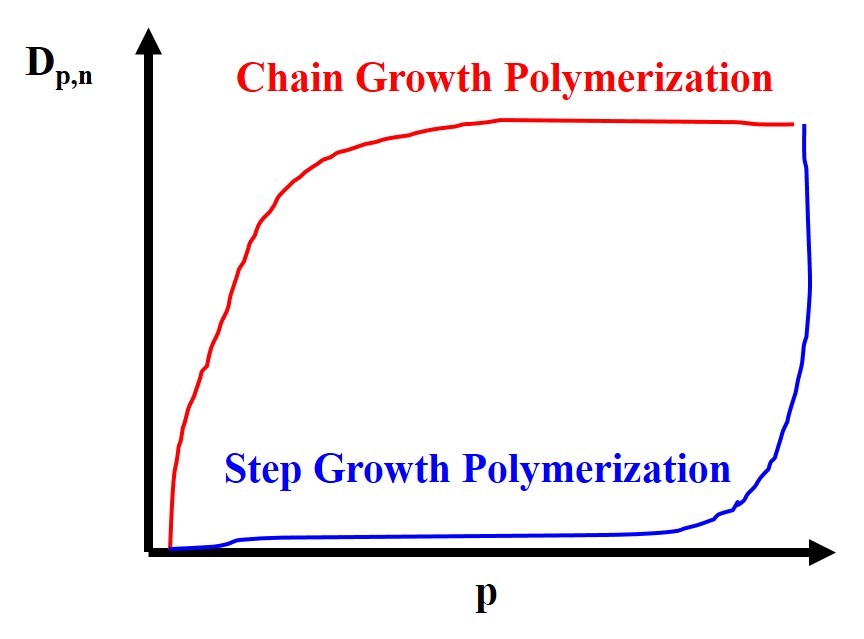
We’ll be briefly discuss several key classes of chain-growth polymerization
• Radical Polymerization
• Cationic Polymerization
• Anionic Polymerization
• Ring Opening Polymerization
• Living Polymerization
• Ziegler-Natta Catalytic Polymerization (HDPE)

Figure 28. Free Radical, Cationic, and Anionic Monomers.
Free Radical Chain Polymerization

So for this method we will need an initiator which is typically a radical and the propagating site is typically a carbon radical. Free radical chain polymerization is particularly adept for polymers that have monomers with C=C double bonds like vinyl, aldehydes, and ketones.
Initiation actually has two steps the creation of free radicals and then the radical are transferred to the monomers. There are a number of different methods to create a free radical including thermal decomposition, photolysis, redox reactions, electrochemistry, sonication, or even ionizing radiation. Once the double bond is broken and the radical is formed the radical initiator will attack the next monomer. The radical is then transferred and propagated. This process continues until the process is terminated or there are no more monomers. This processes is very dependent on experimental conditions, i.e. temperature, solvent, environment, reactivity, etc.
The process is then terminated typically via two methods either combination or radical disproportionality. Combination involves simply the combination of two free radical chains which combine and form a new carbon backbone bond. And disproportionation creates a polymer chain with a saturated carbon bond at the end and another polymer chain with an unsaturated carbon bond at the end of the other polymer chain. There are other termination where an active chain can interact with impurities as well. The basic concept is the radical is converted to a more stable product or products.
The final method to stop polymerization is via chain transfer which involves moving the radical from the end of the chain to another point in the polymer chain. This will typically create branches which is very common in the production of low density polyethylene, LDPE.
Cationic Chain Polymerization
For cationic polymerization the initiator is an acid and the propagating site is a carbocation. The primary monomers for cationic polymerization are olefins and heterocylic monomers (examples). The propagation steps is very similar to the free radical polymerization and the termination step occurs typically via transfer of charge and association with hydrogen or the anion end capping the polymer.
Anionic Chain Polymerization
For anionic polymerization the initiator is a nucleophile the propagating site is a carbanion. The typical monomers are vinyl monomers. Again the propagation step is very similar and the termination step occurs typically via impurities like oxygen or water and the polymerization can be stopped manually by adding water or alcohol.

There are also a number of other polymerization methods like Ring Opening Polymerization. Or Ziegler-Natta Catalytic Polymerization where the initiator is a transition metal complex and the propagating site is a catalytic complex. One additional method to mention is living polymerization where the termination or chain transfer reactions are removed or essentially negligible. This results in a polymerization rate that is very constant/consistent resulting in a very low polydispersity index. This the key difference between HDPE and LDPE.
Again the key thing to take away from this lecture is to understand these different mechanisms, how to produce polymers through a variety of mechanisms and understand via kinetics what reaction will produce the polymer most suited for our application based on PDI and molar mass requirements.