13: Environmental Effects of Forestry
- Page ID
- 83493
\( \newcommand{\vecs}[1]{\overset { \scriptstyle \rightharpoonup} {\mathbf{#1}} } \)
\( \newcommand{\vecd}[1]{\overset{-\!-\!\rightharpoonup}{\vphantom{a}\smash {#1}}} \)
\( \newcommand{\id}{\mathrm{id}}\) \( \newcommand{\Span}{\mathrm{span}}\)
( \newcommand{\kernel}{\mathrm{null}\,}\) \( \newcommand{\range}{\mathrm{range}\,}\)
\( \newcommand{\RealPart}{\mathrm{Re}}\) \( \newcommand{\ImaginaryPart}{\mathrm{Im}}\)
\( \newcommand{\Argument}{\mathrm{Arg}}\) \( \newcommand{\norm}[1]{\| #1 \|}\)
\( \newcommand{\inner}[2]{\langle #1, #2 \rangle}\)
\( \newcommand{\Span}{\mathrm{span}}\)
\( \newcommand{\id}{\mathrm{id}}\)
\( \newcommand{\Span}{\mathrm{span}}\)
\( \newcommand{\kernel}{\mathrm{null}\,}\)
\( \newcommand{\range}{\mathrm{range}\,}\)
\( \newcommand{\RealPart}{\mathrm{Re}}\)
\( \newcommand{\ImaginaryPart}{\mathrm{Im}}\)
\( \newcommand{\Argument}{\mathrm{Arg}}\)
\( \newcommand{\norm}[1]{\| #1 \|}\)
\( \newcommand{\inner}[2]{\langle #1, #2 \rangle}\)
\( \newcommand{\Span}{\mathrm{span}}\) \( \newcommand{\AA}{\unicode[.8,0]{x212B}}\)
\( \newcommand{\vectorA}[1]{\vec{#1}} % arrow\)
\( \newcommand{\vectorAt}[1]{\vec{\text{#1}}} % arrow\)
\( \newcommand{\vectorB}[1]{\overset { \scriptstyle \rightharpoonup} {\mathbf{#1}} } \)
\( \newcommand{\vectorC}[1]{\textbf{#1}} \)
\( \newcommand{\vectorD}[1]{\overrightarrow{#1}} \)
\( \newcommand{\vectorDt}[1]{\overrightarrow{\text{#1}}} \)
\( \newcommand{\vectE}[1]{\overset{-\!-\!\rightharpoonup}{\vphantom{a}\smash{\mathbf {#1}}}} \)
\( \newcommand{\vecs}[1]{\overset { \scriptstyle \rightharpoonup} {\mathbf{#1}} } \)
\( \newcommand{\vecd}[1]{\overset{-\!-\!\rightharpoonup}{\vphantom{a}\smash {#1}}} \)
\(\newcommand{\avec}{\mathbf a}\) \(\newcommand{\bvec}{\mathbf b}\) \(\newcommand{\cvec}{\mathbf c}\) \(\newcommand{\dvec}{\mathbf d}\) \(\newcommand{\dtil}{\widetilde{\mathbf d}}\) \(\newcommand{\evec}{\mathbf e}\) \(\newcommand{\fvec}{\mathbf f}\) \(\newcommand{\nvec}{\mathbf n}\) \(\newcommand{\pvec}{\mathbf p}\) \(\newcommand{\qvec}{\mathbf q}\) \(\newcommand{\svec}{\mathbf s}\) \(\newcommand{\tvec}{\mathbf t}\) \(\newcommand{\uvec}{\mathbf u}\) \(\newcommand{\vvec}{\mathbf v}\) \(\newcommand{\wvec}{\mathbf w}\) \(\newcommand{\xvec}{\mathbf x}\) \(\newcommand{\yvec}{\mathbf y}\) \(\newcommand{\zvec}{\mathbf z}\) \(\newcommand{\rvec}{\mathbf r}\) \(\newcommand{\mvec}{\mathbf m}\) \(\newcommand{\zerovec}{\mathbf 0}\) \(\newcommand{\onevec}{\mathbf 1}\) \(\newcommand{\real}{\mathbb R}\) \(\newcommand{\twovec}[2]{\left[\begin{array}{r}#1 \\ #2 \end{array}\right]}\) \(\newcommand{\ctwovec}[2]{\left[\begin{array}{c}#1 \\ #2 \end{array}\right]}\) \(\newcommand{\threevec}[3]{\left[\begin{array}{r}#1 \\ #2 \\ #3 \end{array}\right]}\) \(\newcommand{\cthreevec}[3]{\left[\begin{array}{c}#1 \\ #2 \\ #3 \end{array}\right]}\) \(\newcommand{\fourvec}[4]{\left[\begin{array}{r}#1 \\ #2 \\ #3 \\ #4 \end{array}\right]}\) \(\newcommand{\cfourvec}[4]{\left[\begin{array}{c}#1 \\ #2 \\ #3 \\ #4 \end{array}\right]}\) \(\newcommand{\fivevec}[5]{\left[\begin{array}{r}#1 \\ #2 \\ #3 \\ #4 \\ #5 \\ \end{array}\right]}\) \(\newcommand{\cfivevec}[5]{\left[\begin{array}{c}#1 \\ #2 \\ #3 \\ #4 \\ #5 \\ \end{array}\right]}\) \(\newcommand{\mattwo}[4]{\left[\begin{array}{rr}#1 \amp #2 \\ #3 \amp #4 \\ \end{array}\right]}\) \(\newcommand{\laspan}[1]{\text{Span}\{#1\}}\) \(\newcommand{\bcal}{\cal B}\) \(\newcommand{\ccal}{\cal C}\) \(\newcommand{\scal}{\cal S}\) \(\newcommand{\wcal}{\cal W}\) \(\newcommand{\ecal}{\cal E}\) \(\newcommand{\coords}[2]{\left\{#1\right\}_{#2}}\) \(\newcommand{\gray}[1]{\color{gray}{#1}}\) \(\newcommand{\lgray}[1]{\color{lightgray}{#1}}\) \(\newcommand{\rank}{\operatorname{rank}}\) \(\newcommand{\row}{\text{Row}}\) \(\newcommand{\col}{\text{Col}}\) \(\renewcommand{\row}{\text{Row}}\) \(\newcommand{\nul}{\text{Nul}}\) \(\newcommand{\var}{\text{Var}}\) \(\newcommand{\corr}{\text{corr}}\) \(\newcommand{\len}[1]{\left|#1\right|}\) \(\newcommand{\bbar}{\overline{\bvec}}\) \(\newcommand{\bhat}{\widehat{\bvec}}\) \(\newcommand{\bperp}{\bvec^\perp}\) \(\newcommand{\xhat}{\widehat{\xvec}}\) \(\newcommand{\vhat}{\widehat{\vvec}}\) \(\newcommand{\uhat}{\widehat{\uvec}}\) \(\newcommand{\what}{\widehat{\wvec}}\) \(\newcommand{\Sighat}{\widehat{\Sigma}}\) \(\newcommand{\lt}{<}\) \(\newcommand{\gt}{>}\) \(\newcommand{\amp}{&}\) \(\definecolor{fillinmathshade}{gray}{0.9}\)After completing this chapter, you will be able to:
- Explain how forest harvesting removes nutrient capital from the site.
- Outline how forestry can damage aquatic ecosystems, and how many of those effects can be avoided.
- Describe how clear-cutting affects biodiversity.
- Explain the special qualities of old-growth forest, and how they are affected by timber harvesting.
- Discuss ecological consequences of the conversion of natural forest into plantations.
- Explain the concept of integrated forest management.
Introduction
Forestry includes both the harvesting of trees and the management of post-harvest succession to foster the regeneration of another forest. Forest science guides these activities by providing an understanding of the environmental factors that affect the productivity of trees. The primary goal of commercial forestry is to provide sustainable harvests of tree biomass that can be used to manufacture lumber, paper, and other industrial products, or as a source of bio-energy. Secondary goals are related to the management of hunted animals (such as deer and fish) on forest landscapes, and sometimes the designation of certain areas as protected (where no timber harvesting occurs).
Forestry is an important economic sector in many countries. This is the case in Canada, where a large industrial enterprise depends on a continuous supply of tree biomass for the production of products that have a great economic value ($34 billion in 2013; see Chapter 14 for forestry-related economics).
Of course, to achieve these economic benefits, trees must be harvested from extensive areas of mature forest. In Canada, clear-cutting is the most commonly used method of forest harvesting – this method removes all of the economically useful trees on a site at the same time. Clear-cutting accounts for about 90% of the annual timber harvest.
However, clear-cutting is not the same as deforestation. Deforestation involves the permanent conversion of a forest into some other kind of ecosystem, such as an agricultural or urbanized land-use. In Canada and most other developed countries, industrial clear-cutting is generally followed by the regeneration of another forest. In fact, it is common practice to manage the post-harvest succession to speed up the rate of regeneration of trees. This allows the next harvest to be made after a relatively short time, so more profit can be made. (This period of time is known as a harvest rotation.) In this sense, forestry as it is usually practised does not result in a net deforestation, and if appropriately managed the forest resource is not depleted. Even though hundreds of thousands of hectares are harvested each year in Canada (638-thousand ha in 2013), the net deforestation is essentially zero (Table 14.11).
Timber harvesting can be viewed as a disturbance of the forest ecosystem, followed by regeneration. Additional disturbances are associated with silvicultural activities, such as preparing the site for planting, thinning dense stands, and applying herbicide or insecticide to deal with pest problems. Silviculture, the branch of forestry that involves tending and growing forests, is practised over an extensive area in Canada. For instance, about 67% of the area harvested (in 2012) is planted to tree seedlings (the other 33% regenerates naturally.) Some planted areas are managed intensively to develop a plantation, which is an anthropogenic forest, but one that lacks many of the ecological and aesthetic values of natural forest.
In this chapter we examine some of the ecological effects of timber harvesting and silviculture, with a focus on site quality, hunted animals, and biodiversity. Additional effects of forestry are examined in other chapters: pesticide spraying in Chapter 22, implications for carbon storage in Chapter 17, and tropical deforestation and global biodiversity in Chapter 26.
Forest Harvesting and Site Capability
In Chapter 14, we defined site capability (or site quality) as the potential of land to sustain the productivity of agricultural crops. This is also relevant to forestry, in terms of the ability of land to sustain the productivity of trees. Site capability is a complex attribute that involves the amounts of nutrients and organic matter in soil, the availability of moisture, and other factors affecting plant growth. These factors are influenced by soil type, climate, drainage, rate of nutrient cycling, and the kinds of plant and microbial communities that are present.
The ability of soil to supply plants with nutrients is a critical aspect of site capability. In large part, this ecological function depends on the nutrient capital of a site, which is the amount of nutrients present in the soil, living vegetation, and dead organic matter. When trees are harvested, the nutrients in their biomass are also removed, which can deplete the nutrient capital of the site.
A stand of forest may be harvested using a variety of methods, which vary in the amount of biomass and nutrients that are removed from the site. A selection harvest is a relatively “soft” method because it involves the harvesting of only some of the trees from a stand, leaving others behind and the structure of the forest substantially intact. The most intensive harvest is a clear-cut, in which all economically useful trees are removed. The smallest clear-cuts, typically involving a hectare or less, are known as a group-selection harvest. More typically, clear-cuts entail the harvesting of trees from larger areas, on the order of 20-100 ha. The largest clear-cuts may extend over hundreds, and even thousands, of hectares. However, such extensive operations are unusual, and are usually associated with the salvaging of trees that have been damaged by a wildfire, windstorm, or insect infestation.
There are also some less intensive methods of clear-cutting. A shelterwood harvest is a staged clear-cut, in which some larger trees of economically desirable species are left standing during the initial cut. These provide a seed source and a partially shaded environment that encourages natural regeneration. Once the regeneration is well underway, the large “leave” trees are harvested. A strip-cut is another kind of staged harvest, in which long and narrow clear-cuts are made at intervals, with uncut forest left in between to provide a source of seed to regenerate trees in the cut strips. Once the regeneration is established, another strip-cut is made, again leaving intact forest on one of the sides. This system of progressive strip-cutting continues until all the forest in the management block (the specific area being managed this way) has been harvested. Typically, an area is harvested in three to four strips. To regenerate trees on the final strips, foresters may rely on advanced regeneration – that is, on small individuals of tree species that existed in the stand prior to harvesting and that survived the disturbance of clear-cutting. Alternatively, they may plant the last strip with seedlings.

Clear-cutting systems also vary in how intensively the biomass of individual trees is harvested. The usual stem-only harvest involves the removal of tree trunks, leaving the roots, stumps, and logging “slash” (cut branches and foliage) on the site. The harvested logs can then be processed into lumber or pulp for manufacturing paper. A whole-tree harvest is more intensive because it removes all of the above-ground biomass of the trees, including the branches and foliage. This intensive kind of harvest will recover considerably more biomass than a stem-only cut, which is an advantage if the wood is to be used as a source of bio-energy.
Nutrient Losses during Harvesting
Although intensive harvests such as a whole-tree clear-cut increases the yield of biomass, there is also considerably more removal of nutrients. Some forest scientists have suggested that the nutrient removals from whole-tree harvests could degrade the capability of sites to sustain tree productivity. The problem would be especially severe if the harvests are conducted over a short rotation. This might not allow enough time for the nutrient capital to recover by natural inputs, such as by precipitation, nitrogen fixation, and weathering of minerals (Figure 23.1).

Site impoverishment caused by intensive cropping is a well-known problem in farming, in which severely degraded land may have to be abandoned for some or all agricultural purposes. However, usually this problem can be managed, to a degree, by applying fertilizer or composted organic matter to the land. Sometimes, however, the degradation of site capability, especially of tilth, is too severe, and this simple mitigation is not successful. Of course, the harvest rotation in agriculture is usually annual, whereas in forestry it ranges from about 20 to 100 years. However, each timber harvest involves the removal of a huge quantity of biomass, and thus of nutrients.
Compare, for example, the amounts of biomass and nutrients removed by clear-cuts of a conifer forest in Nova Scotia (Table 23.1). In this case, a whole-tree clear-cut yielded 30% more biomass than a stem-only harvest. The increased yield may be an advantage, particularly if the harvest is to be used for energy production. The increased harvest of biomass is, however, due to the removal of nutrient-rich tissues such as foliage and small branches. Consequently, the whole-tree harvest removed up to twice as many nutrients as did the stem-only clear-cut. In effect, a 30% increase in biomass yield by the whole-tree method was “purchased” at the ecological “expense” of 54-99% increases in the removal of nutrients.

Unfortunately, there are few studies that allow foresters to compare the productivity of subsequent harvest rotations on the same site. Such studies would take more than 50-100 years, requiring several generations of foresters! Therefore, it is difficult to evaluate the implications of nutrient removal by clear-cutting. Overall, however, it appears that a degradation of site nutrient capital is a less severe problem in forest harvesting than in agriculture. Consequently, nutrient removals by timber harvesting should be viewed as a potential long-term problem. Because forestry is an economically important activity, and the maintenance of site capability is critical to the sustainability of the enterprise, scientists should continue to study the effects of harvesting on nutrient capital. In the short term, however, forestry causes more immediate kinds of damage to site capability and biodiversity that deserve our attention.
Leaching of Nutrients
The disturbance of forested land can increase the rate at which dissolved nutrients are transported downward into the soil with percolating rainwater (a process known as leaching). If the nutrients leach deeper into the soil than tree roots can penetrate, they are effectively lost from the “working” nutrient capital of the site. Eventually, leached nutrients can find their way into groundwater and surface waters.
The nutrients with the greatest tendency to leach are nitrate and potassium, both of which are highly soluble in water. However, calcium, magnesium, and sulphate may also leach in significant amounts. Of course, following a clear-cut, any nutrient losses by leaching are in addition to that removed with tree biomass.
A well-known study of nutrient leaching caused by forest disturbance was done at Hubbard Brook, New Hampshire. This large-scale experiment involved felling all of the trees on a 16-ha watershed, but without removing any biomass – the cut trees were left on the ground. The watershed was then treated with herbicide for three years to suppress regeneration. This experiment was designed to examine the effects of intense disturbance, by de-vegetation, on biological control of watershed functions such as nutrient cycling and hydrology. The research was not intended to examine the effects of a typical forestry practice.
Overall, during a 10-year period following the cutting, the de-vegetated watershed lost 50 kg/ha-year of NO3-N (i.e., nitrogen in the form of nitrate), 45 kg/ha-y of Ca, and 17 kg/ha-y of K in streamflow (Bormann and Likens, 1994; Figure 23.2 shows data for the first three years, which were the most dramatic). The losses were much larger than from an undisturbed reference watershed: 4.3 kg/ha-y of NO3-N, 13 kg/ha-y of Ca, and 2.2 kg/ha-y of K.
In part, the increased losses of nutrients were due to a 31% increase in the yield of water from the de-vegetated watershed (during the first three years after cutting). The increased streamflow was caused by the disruption of transpiration from plant foliage. However, increases in nutrient concentration in the streamwater were more important: during the first three years, NO3 increased by an average factor of 40; K, by 11; Ca, by 5.2; and Mg, by 3.9. The losses of N, Ca, and Mg in the streamwater were similar to their amounts in the above-ground biomass of the forest.
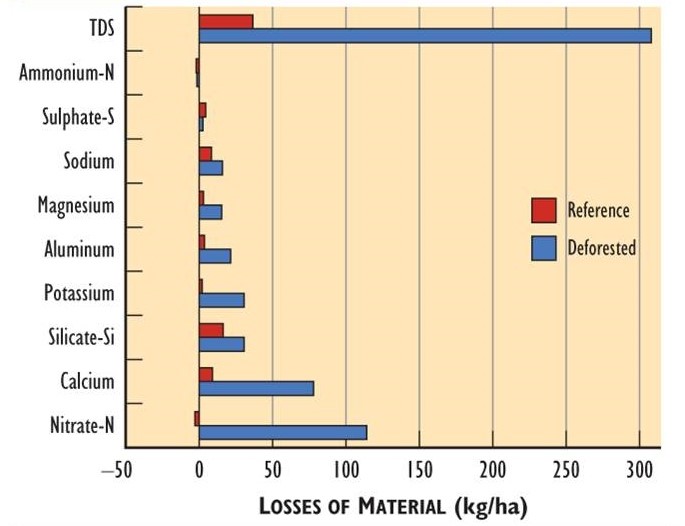
Because this experiment in de-vegetation did not involve a typical forestry practice, the measured effects are unrealistically large. However, watershed-level studies of clear-cutting have also found an increase in nutrient leaching, although to a lesser degree than that caused by the de-vegetation at Hubbard Brook. For example, in the first three years after clear-cutting a 391 ha watershed in New Brunswick, there was an increased loss of nitrate in streamwater of 7 kg NO3-N/ha-yr (Krause, 1982). A study of nine clear-cut watersheds in New Hampshire found an average nitrate loss of 18 kg NO3-N/ha-y during the first four years, compared with 3.5 kg/ha-y for five uncut watersheds (Martin et al., 1986). In addition, calcium losses from the clear-cuts averaged 28 kg/ha-y, compared with 13 kg/ha-y for reference watersheds, while potassium losses were 6 kg/ha-y compared with 2 kg/ha-y. However, other studies have found smaller effects of clear-cutting on nutrient losses with streamflow, especially if only a portion of the watershed was cut.
Nitrate and other highly soluble ions are leached from watersheds after clear-cutting (and after other disturbances, such as wildfire) for several reasons. First, disturbance stimulates the activity of microbes involved in the decomposition of organic matter. This occurs because removal of the tree canopy results in warming of the forest floor and surface soil, and decreased uptake by plants leads to an increase of soil nutrients and moisture. Second, disturbance often stimulates the microbial processes of ammonification and nitrification (see Chapter 5), resulting in increased rates of production of nitrate, which is extremely soluble and readily lost from soil.
Forestry and Erosion
Forestry activities can cause severe losses of soil, or erosion, particularly in terrain with steep slopes. In most cases, erosion is triggered by improperly constructing logging roads, using streams as trails to haul logs, running log-removal trails down slopes instead of along them, and harvesting trees from steep slopes that are extremely vulnerable to soil loss. In general, however, road building is the most important cause of erosion on forestry lands, especially where culverts (channelled stream crossings) are not sufficiently large or numerous, or are poorly installed.
Severe erosion causes many environmental damages. In extreme cases, the loss of soil may expose bedrock, making forest regeneration impossible. Soil loss also represents a depletion of site nutrient capital. Erosion also causes secondary damage to aquatic habitats, including the deposition of silt (or siltation), which covers gravel substrates that are important to spawning fish. Also, the shallower water increases the risk of flooding.
However, in many cases erosion is a largely avoidable environmental effect of forestry. The irresponsible practices that can cause erosion are restricted by provincial regulations and occur much less frequently now than in the past. Practices that help to reduce erosion include the following:
- planning the route of forest roads to avoid stream crossings as much as possible
- installing a sufficient number of properly sized culverts
- avoiding the disturbance of stream channels by heavy equipment
- leaving buffer strips of uncut forest beside watercourses
- using log-removal practices that avoid disturbance of the forest floor (such as cable yarding, in which a tall spar anchors cables radiating into the clear-cut, which allows logs to be dragged to a central place without the use of a wheeled skidder)
- allowing vegetation to regenerate quickly, which speeds the re-establishment of biological moderation of erosion
- deciding to selectively harvest, or to not harvest, steep sites that are highly vulnerable to erosion
It has become a common practice to leave strips of uncut forest beside streams, rivers, and lakes. These buffer zones greatly reduce the erosion of streambanks, eliminate temperature increases in the water, maintain riparian (lake- and stream-side) habitat for wildlife, and mitigate some of the aesthetic damage from forest harvesting.
While it is widely accepted that riparian buffers provide important benefits, there is no consensus about how wide the uncut strips should be. This is an economically important consideration, because large areas of valuable timber are withdrawn from the potential harvest when buffer strips are left. The requirements in New Brunswick, for example, are for a 30-m buffer on each side of a watercourse, with wider buffers recommended in some circumstances (such as 60 m if the slope exceeds 24°, and up to 100 m beside waters that are used for recreation or as a source of drinking water).
In some cases, selective harvesting of trees may be allowed within riparian buffers, as long as this does not compromise the ecological services provided by these special management zones.
Forestry and Hydrology
The cover of forest on a watershed has a strong influence on its hydrology. Large amounts of water are evaporated into the atmosphere by vegetation, especially by trees because they have so much foliage (this is transpiration; evapotranspiration includes evaporation from non-living surfaces). In the absence of transpiration, an equivalent quantity of water would leave the watershed as streamflow or as seepage to deeper groundwater.
For example, studies of four well-forested watersheds in Nova Scotia found that evapotranspiration was equivalent to 15-29% of the annual input of water by precipitation (rain plus snow), with runoff in streams accounting for the other 71-85% (these watersheds have no substantial drainage to deep groundwater; Freedman et al., 1985).
The hydrologic budget of watersheds is extremely seasonal, particularly in the temperate and boreal climates that are typical of forested regions of Canada. This can be illustrated by the watershed of the Mersey River in Nova Scotia (Figure 23.3). The annual input of water from precipitation was 146 cm/y, with 82% arriving as rain and 18% as snow. About 62% of the annual input was dispersed by riverflow and 38% by evapotranspiration. Evapotranspiration is highest during the growing season, which results in relatively sparse riverflow. Runoff is greater during late autumn and early winter, when there is little transpiration because deciduous trees have dropped their foliage, and even conifers are in a quiescent state. However, much of the precipitation during that period recharges groundwater storage, which had been depleted by the uptake of water by vegetation during the summer. Runoff is greatest during the spring, when the accumulated snowpack melts, and that results in a spate of riverflow.
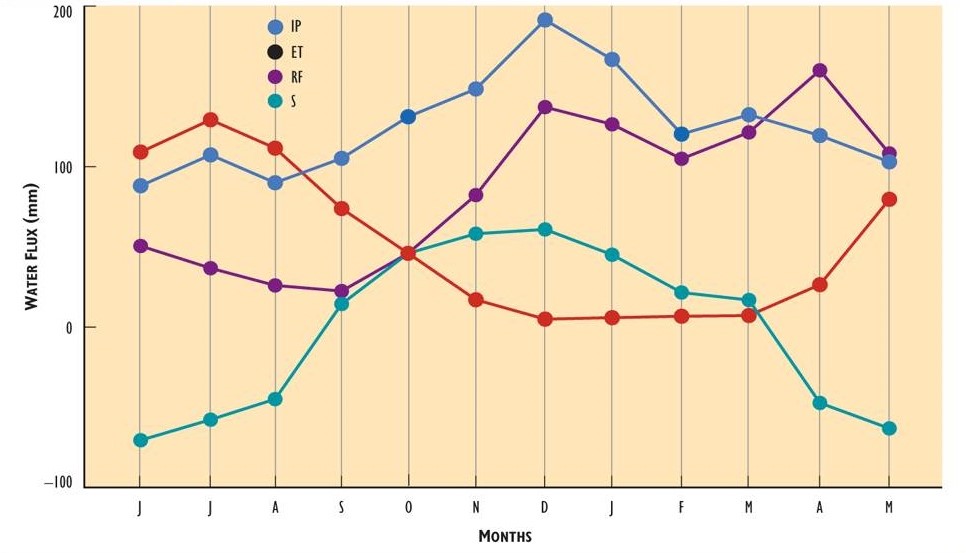
Disturbances such as wildfire and timber harvesting alter the hydrology of watersheds. The seasonality and amounts of flow can change, and erosion and flooding may occur downstream. Some poorly drained sites may become wetter, because reduced transpiration can raise the water table. In general, the increase in streamflow is related to the proportion of the watershed that was disturbed. If an entire watershed is clear-cut, the increase in streamflow can be as much as 40% in the first year. The increase is proportionately less after partial cuts.
Clear-cuts usually regenerate quickly, and in some cases the vigorous regrowth of shrubs and herbs can restore most of the original foliage area in as few as four to six years. Consequently, the biggest increases in streamflow occur in the first year after cutting, followed by rapid recovery to the pre-harvest condition. In the temperate and boreal climates prevalent in much of Canada, the largest increases in streamflow occur during the late spring, summer, and early autumn, these being the seasons when transpiration is most important.
Hydrology can also be affected by a change in the type of forest that is dominant on a watershed. For example, if an area of hardwood forest is converted into conifer plantations, the annual streamflow may decrease. This happens because the conifers maintain their foliage throughout the year, and so extend the transpiration season into times when angiosperm trees lack foliage.
Weeds and Reorganization
Clear-cuts usually regenerate rather quickly. Initially, however, most of the regenerating biomass involves plants other than the tree species that foresters consider desirable. As a result, the vigorous regrowth is often regarded as being detrimental to silvicultural objectives. Such non-crop plants may be viewed as “weeds,” and their abundance may be controlled by a herbicide application (see Chapter 22).
However, a rapid re-vegetation of clear-cuts and other disturbed lands does provide important ecological benefits. The regenerating plants influence the ecological “reorganization” of disturbed lands. They re-establish a measure of biological control over nutrient cycling, erosion, and hydrology, while also restoring habitat for animals.
For example, during the first few years after clear-cutting, fast-growing vegetation restores a high rate of nutrient uptake from the soil. Through this uptake, the regenerating vegetation acts as a “sponge” that absorbs some of the soluble nutrients that might otherwise leach from the site. Eventually, the early successional plants die, and their nutrients are recycled by decomposition and made available to trees. In addition, the re-vegetation restores habitat for birds, mammals, and other wildlife. Clearly, the early reorganization phase of succession is enhanced by the rapid regeneration of many plant species, including those considered to be weeds by foresters.
Forestry and Biodiversity
Clear-cutting and other forestry practices inflict intense disturbances on forests. They cause dramatic changes in the habitat available to support plants, animals, and microbes, as well as their various communities. Some species benefit from habitat changes that occur because of forestry, but others suffer damage.
In the following sections, we examine the effects of forestry on aspects of Canadian biodiversity – the richness of biological variation in our country. The effects of clear-cutting on plants, mammals, birds, and fish will be examined because these groups are relatively well studied and are considered to be important by our society.
Vegetation
Any severe disturbance results in changes in the dominant species of plants that are living on a site. Because they have such a great influence on local environmental conditions, trees are the dominant organisms in forests. After clear-cutting, many smaller plants take advantage of the relatively uncompetitive conditions that occur, and they dominate the initial stages of the post-harvest succession. They are then reduced in abundance, or even eliminated from the community, once several decades of regeneration have gone by and tree-sized plants re-establish their dominance.
Many plants of early post-cutting succession can only be successful in open habitats –they are intolerant of the shade and other stressful conditions beneath a forest canopy. These relatively short-lived ruderal plants can typically disperse widely, a propagation strategy made necessary because of their ephemeral habitat (see Chapter 9). Ruderal plants that can proliferate in recently disturbed forests include asters, goldenrods, grasses, and sedges. A specific example is the fireweed (Chamerion angustifolium), a purple-flowered herb that is often abundant after wildfire (hence its name) and also after clear-cutting. Some woody plants are also ruderals, because they are most abundant during the recovery after disturbance. Examples are the red raspberry (Rubus strigosus), pin cherry (Prunus pensylvanica), and elderberry (Sambucus racemosa). Because of their need for open, recently disrupted habitat, ruderal plants benefit from clear-cutting and other disturbances that are associated with forestry.
Unlike ruderal plants, some other species are tolerant of the environmental stress that occurs beneath a closed forest canopy. Examples are the white trillium (Trillium grandiflorum), shield fern (Dryopteris marginalis), feather mosses (such as Pleurozium schreberi and Hylocomium splendens), and certain lichens, such as lungwort (Lobaria pulmonaria). These species are not tolerant of open conditions, and they decline after clear-cutting. Once suitable conditions re-develop, these plants may again increase in abundance.
In general, once a clear-cut has had two to four years to regenerate, the plant community is actually richer in species than the mature forest that was harvested (this is particularly true of vascular plants). The increase in species diversity occurs because recently disturbed habitats are relatively flush in such resources as light, nutrients, and water. This allows many species of low-growing plants to be supported, including a diversity of ruderal ones. In comparison, stressful habitats, such as the understorey beneath a mature forest canopy, support fewer species of plants.
A study of a hardwood forest in Nova Scotia illustrates the species-rich nature of vegetation after a clear-cut (Crowell and Freedman, 1994). That study examined stands of mature forest, plus clear-cuts of various age. The number of plant species in the ground vegetation (shorter than 2 m) averaged 11/m2 on two one-year-old clear-cuts, and increased to 14/m2 on six-year-old clear-cuts. Mature forest and clear-cuts older than 30 years had fewer species – only 3-6/m2 in stands with a closed canopy dominated by maple trees, but 9-11/m2 in birch-dominated stands, which have a more open canopy. This comparison suggests that many plants, especially ruderals, can utilize open habitats associated with clear-cutting. However, species that need mature forest as habitat are threatened by this kind of disturbance.
Conclusions
Forestry is a key economic sector in Canada, each year affecting millions of hectares of landscape. Timber harvesting and management of the subsequent regeneration cause many environmental changes, including decreases of carbon storage, alterations of hydrology, erosion, and effects on the habitat of wildlife. Because timber harvesting and silvicultural practices are severe disturbances of forested sites, some environmental damage is inevitable. To a large degree, however, many of the damages could be mitigated by adopting different forestry practices than are currently used. These include less use of the predominant clear-cutting system, and replacing it with softer practices such as selection harvesting. Greater attention to the protection of aquatic habitat is also necessary, such as retaining buffer strips of uncut forest along all watercourses. Some damage to the habitat of wildlife on cutovers can also be mitigated, for instance, by retaining cavity trees and by greater reliance on natural regeneration rather than on plantations. It is also necessary to protect large areas of natural forest from intensive resource harvesting. This requires the implementation of a connected network of protected areas that is sufficient to conserve those species and ecological communities that are incompatible with use of the landscape for forestry purposes.
Questions for Review
- How do forestry practices threaten the nutrient capital and site quality of harvested stands?
- How does timber harvesting affect the hydrology of streams and rivers?
- What elements should an integrated management plan include for a typical forested watershed in your region? Consider the needs to ensure a constant supply of timber, deer, sportfish, clean water, and habitat for non-game species.
- What are the characteristics of a typical old-growth forest in the region where you live? Do you think that the special values of the old-growth forest can be accommodated by forestry, or can old-growth forest be preserved only by creating large protected areas where trees are not harvested? What are the economic implications of setting aside such large tracts of potentially valuable timber? What are the ecological implications?
Questions for Discussion
- Consider a typical forest in the region where you live. What are the dominant species of trees, other plants, and animals that live in that forest? What are some important interactions among those species? Consider, for example, the habitat needs of certain animals, including their foods.
- Are forest products important in your life and in the functioning of your community? Compile a list that shows how trees are used for bio-energy, lumber, paper, and other products. Also consider non-timber uses of forests, such as hunted animals, recreation, carbon storage, and the provision of clean air and water.
- How might forest resources make a larger contribution to the Canadian economy, or to that of your region? Would it be possible, for example, to harvest more wood without degrading the timber resource? Could more people be employed in woodland operations if harvesting and management activities were less mechanized? Consider also the prospects for reducing the export of raw materials, such as logs, through increased local processing into manufactured products.
- Why is it essential that a large and connected system of protected areas be a key part of any landscape-scale plan for ecologically sustainable forestry?
Exploring Issues
- A proposal is being made to build a lumber mill in an area that is now wilderness. About 40% of the forest in the area is in an old-growth condition. You are an ecologist, working as part of a team of scientists to assess the potential environmental impacts of the forest management plan to supply timber to the proposed sawmill. Your responsibility is to consider the sustainability of the supply of timber and other forest resources, as well as effects on rare species and natural ecosystems. What would you examine to ensure that the forest management is ecologically sustainable?
References Cited and Further Reading
Barnes, B.V., S. Spurr, D. Zak, and S. Denton. 1998. Forest Ecology. 4th ed. John Wiley & Sons, New York, NY.
Berger, J.J. 1998. Understanding Forests. Sierra Club, San Francisco, CA.
Bormann, F.H. and G.E. Likens. 1994. Pattern and Process in a Forested Ecosystem. Springer, New York, NY.
Boyce, M.S. and A. Haney (eds.). 1999. Ecosystem Management: Applications for Sustainable Forest and Wildlife Resources. Yale University Press, New Haven, CT.
Buskirk, S.W. 1992. Conserving circumboreal forests for martens and fisher. Conservation Biology, 6: 318-320.
Crowell, M. and B. Freedman. 1994. Vegetation development during a post-clearcutting chronosequence of hardwood forest in Nova Scotia, Canada. Canadian Journal of Forest Research, 24: 260-271.
Drolet, C.A. 1978. Use of forest clear-cuts by white-tailed deer in southern New Brunswick and central Nova Scotia. Canadian Field-Naturalist, 92: 275-282.
Freedman, B. 1995. Environmental Ecology. 2nd ed. Academic Press, San Diego, CA.
Freedman, B., R. Morash, and A.J. Hanson. 1981. Biomass and nutrient removals by conventional and whole-tree clear-cutting of a red spruce-balsam fir stand in central Nova Scotia. Canadian Journal of Forest Research, 11: 249-257.
Freedman, B., C. Stewart, and U. Prager. 1985. Patterns of Water Chemistry of Four Drainage Basins in Central Nova Scotia. Technical Report IWD-AR-WQB-85-93, Environment Canada Moncton, NB.
Freedman, B., S. Woodley, and J. Loo. 1994. Forestry practices and biodiversity, with particular reference to the Maritime Provinces of eastern Canada. Environmental Reviews, 2: 33-77.
Freedman, B., V. Zelazny, D. Beaudette, T. Fleming, S. Flemming, G. Forbes, G. Johnson, and S. Woodley. 1996. Biodiversity implications of changes in the quantity of dead organic matter in managed forests. Environmental Reviews, 4: 238-265.
Friends of Clayoquot Sound. 2014. About Clayoquot Sound. www.focs.ca/ Gillis, A.M. 1990. The new forestry: an ecosystem approach to land management. BioScience, 40: 558-562.
Gullion, G.W. 1988. Aspen management for ruffed grouse. Pp. 9-12 in: Integrating Forest Management for Wildlife and Fish. General Technical Report NC-122, North Central Forest Experiment Station, St. Paul, MN.
Harris, L.D. 1984. The Fragmented Forest. University of Chicago Press, Chicago, IL.
Hunter, M.L. 2003. Wildlife, Forests, and Forestry: Principles of Managing Forests for Biological Diversity. Prentice Hal0,l Englewood Cliffs, NJ.
Johnson, G.A.M. and B. Freedman. 2002. Breeding birds in forestry plantations and natural forest in the vicinity of Fundy National Park, New Brunswick. Canadian Field-Naturalist, 116: 475-487.
Kimmins, J.P. 2003. Forest Ecology: A Foundation for Sustainable Management. 2nd ed. Upper Saddle River, NJ: Prentice Hall College Division.
Kimmins, J.P. 2005. Forest Ecology. 3rd ed. Macmillan, New York, NY.
Kimmins, J.P. 1997. Balancing Act: Environmental Issues in Forestry. 2nd ed. University of British Columbia Press, Vancouver, BC.
Krause, H.H. 1982. Nitrate formation and movement before and after clear-cutting of a monitored watershed in central New Brunswick. Canadian Journal of Forest Research, 12: 922-930.
Likens, G.E. and F.H. Bormann. 1999. Biogeochemistry of a Forested Ecosystem. 2nd ed. Springer, New York, NY.
Martin, C.W., R.S. Pierce, G.E. Likens, and F.H. Bormann. 1986. Clearcutting Affects Stream Chemistry in the White Mountains of New Hampshire. NE-579, Northeastern Forest Experiment Station, USDA Forest Service, Broomall, PA.
Maser, C. 1990. The Redesigned Forest. Stoddart, Toronto.
Maser, C. 2001. Forest Primeval: The Natural History of an Ancient Forest. Oregon State University Press, Corvallis, OR.
Maser, C., A.W. Claridge, and J.M. Trappe. 2007. Trees, Truffles and Beasts: How Forests Function. Rutgers University Press, Rutgers, NJ.
McRae, D.J., L.C. Duchesne, B. Freedman, T.J. Lynham, and S. Woodley. 2001. Differences between wildfire and clearcutting and their implications in forest management. Environmental Reviews, 9: 223-260.
Morgan, K. and B. Freedman. 1986. Breeding bird communities in a hardwood forest succession in Nova Scotia. Canadian Field-Naturalist, 100: 506-519.
Smith, D.M., B.C. Larson, M.J. Kelty, and P.M.S. Ashton. 1996. The Practice of Silviculture: Applied Forest Ecology, 9th Ed. J. Wiley and Sons, New York, NY.
Swan, D., B. Freedman, and T. Dilworth. 1984. Effects of various hardwood forest management practices on small mammals in Nova Scotia. Canadian Field-Naturalist, 98: 362-364.
Swanson, F.J. and J.F. Franklin. 1992. New forestry principles from ecosystem analysis of Pacific Northwest forests. Ecological Applications, 2: 262-274.
Welsh, D. and D.R. Fillman. 1980. The impact of forest cutting on boreal bird populations. American Birds, 34: 84-94.
World Resources Institute. 2008. Earth Trends. The Environmental Information Portal. Washington, DC. http://www.wri.org/