Conductive Polymers
- Page ID
- 349
\( \newcommand{\vecs}[1]{\overset { \scriptstyle \rightharpoonup} {\mathbf{#1}} } \)
\( \newcommand{\vecd}[1]{\overset{-\!-\!\rightharpoonup}{\vphantom{a}\smash {#1}}} \)
\( \newcommand{\id}{\mathrm{id}}\) \( \newcommand{\Span}{\mathrm{span}}\)
( \newcommand{\kernel}{\mathrm{null}\,}\) \( \newcommand{\range}{\mathrm{range}\,}\)
\( \newcommand{\RealPart}{\mathrm{Re}}\) \( \newcommand{\ImaginaryPart}{\mathrm{Im}}\)
\( \newcommand{\Argument}{\mathrm{Arg}}\) \( \newcommand{\norm}[1]{\| #1 \|}\)
\( \newcommand{\inner}[2]{\langle #1, #2 \rangle}\)
\( \newcommand{\Span}{\mathrm{span}}\)
\( \newcommand{\id}{\mathrm{id}}\)
\( \newcommand{\Span}{\mathrm{span}}\)
\( \newcommand{\kernel}{\mathrm{null}\,}\)
\( \newcommand{\range}{\mathrm{range}\,}\)
\( \newcommand{\RealPart}{\mathrm{Re}}\)
\( \newcommand{\ImaginaryPart}{\mathrm{Im}}\)
\( \newcommand{\Argument}{\mathrm{Arg}}\)
\( \newcommand{\norm}[1]{\| #1 \|}\)
\( \newcommand{\inner}[2]{\langle #1, #2 \rangle}\)
\( \newcommand{\Span}{\mathrm{span}}\) \( \newcommand{\AA}{\unicode[.8,0]{x212B}}\)
\( \newcommand{\vectorA}[1]{\vec{#1}} % arrow\)
\( \newcommand{\vectorAt}[1]{\vec{\text{#1}}} % arrow\)
\( \newcommand{\vectorB}[1]{\overset { \scriptstyle \rightharpoonup} {\mathbf{#1}} } \)
\( \newcommand{\vectorC}[1]{\textbf{#1}} \)
\( \newcommand{\vectorD}[1]{\overrightarrow{#1}} \)
\( \newcommand{\vectorDt}[1]{\overrightarrow{\text{#1}}} \)
\( \newcommand{\vectE}[1]{\overset{-\!-\!\rightharpoonup}{\vphantom{a}\smash{\mathbf {#1}}}} \)
\( \newcommand{\vecs}[1]{\overset { \scriptstyle \rightharpoonup} {\mathbf{#1}} } \)
\( \newcommand{\vecd}[1]{\overset{-\!-\!\rightharpoonup}{\vphantom{a}\smash {#1}}} \)
\(\newcommand{\avec}{\mathbf a}\) \(\newcommand{\bvec}{\mathbf b}\) \(\newcommand{\cvec}{\mathbf c}\) \(\newcommand{\dvec}{\mathbf d}\) \(\newcommand{\dtil}{\widetilde{\mathbf d}}\) \(\newcommand{\evec}{\mathbf e}\) \(\newcommand{\fvec}{\mathbf f}\) \(\newcommand{\nvec}{\mathbf n}\) \(\newcommand{\pvec}{\mathbf p}\) \(\newcommand{\qvec}{\mathbf q}\) \(\newcommand{\svec}{\mathbf s}\) \(\newcommand{\tvec}{\mathbf t}\) \(\newcommand{\uvec}{\mathbf u}\) \(\newcommand{\vvec}{\mathbf v}\) \(\newcommand{\wvec}{\mathbf w}\) \(\newcommand{\xvec}{\mathbf x}\) \(\newcommand{\yvec}{\mathbf y}\) \(\newcommand{\zvec}{\mathbf z}\) \(\newcommand{\rvec}{\mathbf r}\) \(\newcommand{\mvec}{\mathbf m}\) \(\newcommand{\zerovec}{\mathbf 0}\) \(\newcommand{\onevec}{\mathbf 1}\) \(\newcommand{\real}{\mathbb R}\) \(\newcommand{\twovec}[2]{\left[\begin{array}{r}#1 \\ #2 \end{array}\right]}\) \(\newcommand{\ctwovec}[2]{\left[\begin{array}{c}#1 \\ #2 \end{array}\right]}\) \(\newcommand{\threevec}[3]{\left[\begin{array}{r}#1 \\ #2 \\ #3 \end{array}\right]}\) \(\newcommand{\cthreevec}[3]{\left[\begin{array}{c}#1 \\ #2 \\ #3 \end{array}\right]}\) \(\newcommand{\fourvec}[4]{\left[\begin{array}{r}#1 \\ #2 \\ #3 \\ #4 \end{array}\right]}\) \(\newcommand{\cfourvec}[4]{\left[\begin{array}{c}#1 \\ #2 \\ #3 \\ #4 \end{array}\right]}\) \(\newcommand{\fivevec}[5]{\left[\begin{array}{r}#1 \\ #2 \\ #3 \\ #4 \\ #5 \\ \end{array}\right]}\) \(\newcommand{\cfivevec}[5]{\left[\begin{array}{c}#1 \\ #2 \\ #3 \\ #4 \\ #5 \\ \end{array}\right]}\) \(\newcommand{\mattwo}[4]{\left[\begin{array}{rr}#1 \amp #2 \\ #3 \amp #4 \\ \end{array}\right]}\) \(\newcommand{\laspan}[1]{\text{Span}\{#1\}}\) \(\newcommand{\bcal}{\cal B}\) \(\newcommand{\ccal}{\cal C}\) \(\newcommand{\scal}{\cal S}\) \(\newcommand{\wcal}{\cal W}\) \(\newcommand{\ecal}{\cal E}\) \(\newcommand{\coords}[2]{\left\{#1\right\}_{#2}}\) \(\newcommand{\gray}[1]{\color{gray}{#1}}\) \(\newcommand{\lgray}[1]{\color{lightgray}{#1}}\) \(\newcommand{\rank}{\operatorname{rank}}\) \(\newcommand{\row}{\text{Row}}\) \(\newcommand{\col}{\text{Col}}\) \(\renewcommand{\row}{\text{Row}}\) \(\newcommand{\nul}{\text{Nul}}\) \(\newcommand{\var}{\text{Var}}\) \(\newcommand{\corr}{\text{corr}}\) \(\newcommand{\len}[1]{\left|#1\right|}\) \(\newcommand{\bbar}{\overline{\bvec}}\) \(\newcommand{\bhat}{\widehat{\bvec}}\) \(\newcommand{\bperp}{\bvec^\perp}\) \(\newcommand{\xhat}{\widehat{\xvec}}\) \(\newcommand{\vhat}{\widehat{\vvec}}\) \(\newcommand{\uhat}{\widehat{\uvec}}\) \(\newcommand{\what}{\widehat{\wvec}}\) \(\newcommand{\Sighat}{\widehat{\Sigma}}\) \(\newcommand{\lt}{<}\) \(\newcommand{\gt}{>}\) \(\newcommand{\amp}{&}\) \(\definecolor{fillinmathshade}{gray}{0.9}\)Polymers are organic macromolecules, a long carbonic chain, composed by structural repeat entities, called mer. These smallest unit, for instance, are bonded by covalent bonds, repeating successively along a chain. A monomer, molecule composed by one mer, is the raw material to produce a polymer. The majority of polymers are insulators, due to an unavailability of free electrons to create the conductivity. In a covalent bond, showed in figure 1, the electrons are locked in these strong and directional bonds(see more at [a] of "Links" Section), so when an electric field is applied, electrons cannot drift. Therefore, these type of materials do not show a high conductivity.
The poor conductivity of polymers is also explained by the band theory (see more at [b] of "Links" Section). This theory says that the energy levels of electrons can occupy are grouped in allowed bands and may have energy levels of electron that are forbidden, denominated band gap, as shown in figure 2. This theory is resulted of Schrödinger’s Equation applied in a periodic field of a crystal solid. The lowest bands are called valence bands and are inert on an electrical perspective. On the other hand, the highest bands, which participate in the electric conduction, are called conduction bands.
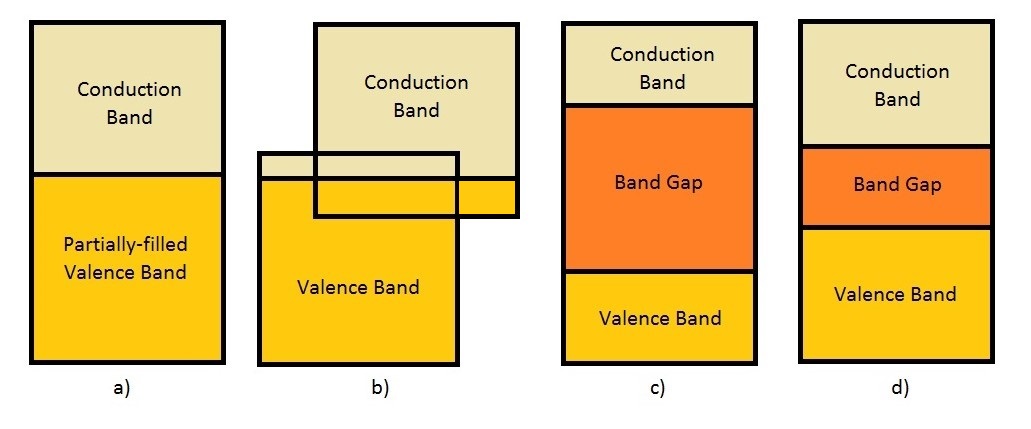
Some conductors has a partially filled valence band that is relatively easier to excite an electron to a higher energy level, as in a). Other conductors, such as divalent metals represented in b), can have an overlap of the empty conductive band with a totally filled valence band. For semiconductor and insulators, represented in d) and c) respectively, the valence electrons must cross the band gap in order to result a conduction. The difference is a semiconductor has a relatively smaller band gap energy than insulators have . For a long period of time, polymers were considered as insulators. Until, in 1970, when the first intrinsic conductive polymer was produced by Shirakawa, Heeger, and MacDiarmid, which resulted in the Nobel prize in 2000. The polymer was produced by the exposure of the polyacetylene to dopant compounds: oxidizing or reducing agents; electron-donor or electron-receptor of electrons.
Conductive Polymers
An electric current results of the orderly movement of charges in a material as a response of forces that act on them, when a voltage is applied. The positive charges flow in the direction of the electric field applied, whereas the negative charges move in the opposite direction. In the majority of materials, a current is resulted by the flux of electrons, known as electric conduction (see more at [c] of "Links" Section).
The structure of these materials has conjugated chains, that is, an alternating single and double bond between the atoms. The process of doping of conductive polymers becomes easier due to these conjugated bonds. In this process, defects and deformations in the polymeric chain are formed. An electron-deformation pair, or also an electron-phonon cloud pair, is called polarons, which is responsible by the conductivity in polymers. Bipolarons and solitons, other types of quasi-particles, also participates in the conductivity mechanism. The type of soliton, bipolaron, or polaron formed depends on the dopant used. Their meaning and the physics behind that is beyond the scope of this subject.
The charges resulted the doping process in conductive polymers is the reason of their great conductivity. The constant movement of the double bonds to stabilize the charge in the neighbor atoms causes, therefore, the movement of the charge, resulting in the conductivity. This movement of double bonds is called resonance (see more at [d] of "Links" Section) and it describes the delocalized electrons within a molecule. A delocalized electron is an electron, presented in a π bond, which is shared by three or more atoms (see more at [e] of "Links" Section). Due to this process of the polaron formation, there is a change in the band structure of the conductive polymer. It creates the polaronic conduction bands, allowed bands in the band gap, reducing the band gap energy, making the polymer able to conduct, as shown in figure 3.
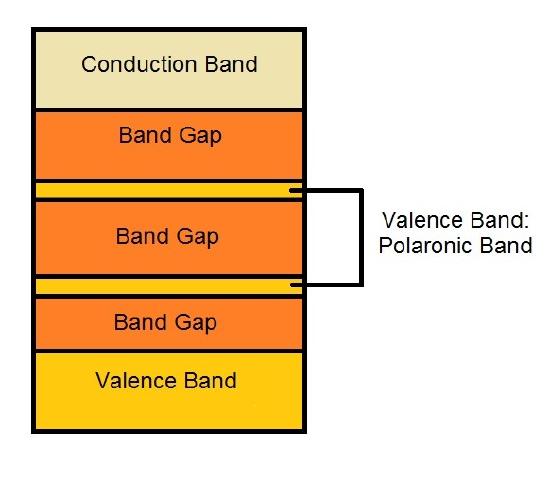
Since the conductivity of a conductive polymer is due to the charge formed by the dopant, as the doping level increases, more charges are formed in the polymer and, thus, results in a greater conductivity. The conductivity of a conductive polymer is also temperature dependent, because as the temperature increases the molecules becomes farther from each other. Thus, the doping effect is more effective and, consequently, the amount of charges, which is the doping level of the polymer is greater, increasing the conductivity. Moreover, as the temperature increases, the energy of an electron is related with the temperature by the Boltzmann relationship. Because of that, the greater the temperature, the greater is the energy of the electrons, and, consequently, the easier is to excite the electron to the conduction band. The best-known and most studied conductive polymers are polyacetilene, polyaniline, and polypyrrole due to their wide range of applications.
Polyacetilene
Polyacetilene is one of the polymers in the study that resulted in the Chemistry Nobel prize in 2000. The polymer is synthetized by the reaction of the etyne, as commercially known as acetylene, with Ziegler-Natta catalyst. The resulting structure is shown below.
The polyacetilene is the simplest polymer that shows a great value of conductivity. The anternating double and single bond in the polymer structure is what gives the polymer the ability of resonate. As dopants are inserted in the polymer, the chains of polymers presents charges. The movement of these charge, by resonance when a field is applied, gives rise to the conductivity of the material.
Polyaniline
Polyaniline is one of the most promising conductive polymers and, therefore, one of the most studied ones. This polymer, in fact, is a family of polymers that is classified by aromatic rings bonded together by nitrogen atoms. Its structure is composed by x units of reduced species alternated with 1-x units of oxidized species, as observed in figure 6.
The doping process occurs through the protonation of the nitrogen atoms in the amine groups of the polyaniline by an acidic solution, oxidizing the structure. Thus, positive charges are formed in the polyaniline structure that moves as the structure resonates, shown in figure 7.
Polypyrrole
The polypyrrole is one of the conductive polymers studied in the research that resulted in the Nobel prize. It is obtained by the oxidization of the pyrrole, resulting in the structure shown below.
The Redox process of the addition of an anion to the structure that is responsible to the conductive properties of the polypyrrole. Again here, the resonance occurs to stabilize the carbocation, moving the charge, resulting in conductivity, mechanism similar as shown for the previous polymers.
Synthesis
There are three ways to produce conductive polymers: reactional chemistry, electrochemical, and photoelectrochemical, being the first one the most used, due to its high profitability and efficiency.
The chemical process consists in the union of monomers by the addition of the oxidizing or reducing agents that makes the neutral polymer to a cationic or anionic ionic complex, ending the reaction by the bonding of this complex to the counter-ion of the redox agent. This process requires a high control, since the reaction is very exothermic and emits gases, requiring proper treatment and equipment of protection.
The electrochemical method consists in the electronic deposition of the polymer in the electrode. The solution that the electrode is immersed has the monomers and the dopants. When a voltage or a current strong enough is applied, the monomers oxidize resulting in the polymerization. This process results in polymers with shape of the electrode, requiring a posterior processing to get the shape desired.
The photoeletrochemical process is based on photoexcitation of the polymer or in compounds that have catalyst properties in presence of light, oxidizating the monomers resulting in a polymerization. Even though this process is simple and environmentally friendly, the mechanical properties of the resulting polymer is not good.
Applications
This type of materials has generated interesting in many field of study, due to its conductivity properties as a metals, but remaining its relatively inert and good mechanical properties. There are many applications of these materials in electronics, such as batteries, sensors, and microelectronics devices. The polypyrrole and polyaniline are currently used in protection of metals, as an anti-corrosive coating. In the medical field, the conductive polymers can be used in the production artificial muscles, biosensors, and drugs controlled-release agents.
Future Prospects and Development of Research
The conductive polymers have a widely range of application, but in some of them the use of the metals is the better option. This is due to their high conductivity obtained by a cheaper process. Thus, the application of these materials will occur where their differential properties are useful, such as the weightless properties of polymers and their application in electronics will result in increasingly smaller and compact devices. The shielding of the electromagnetic interference and radiofrequency is also a likely market where the conductive polymers can have an easier acceptability. With the development of muscles using conductive polymers, the research about its biocompatibility will be promising in the future. The scientists also investigates the effects of doping levels, temperature and band gap in the material in order to determine the best levels to get the better conductive polymer.
Questions
- What are the three method of production of conductive? And what are their limitations?
- Why do most of polymers have a poor conductivity? And why do a conductive polymer have a great conductivity? [Hint: Your answer must have this words: covalent bond, delocalized electrons, resonance, dopants.]
- Draw the band structure of a polymer and a conductive polymer. Discuss what results this difference
- Circle or complete the blanks
a)Polymers are macro/micro molecules composed mostly by ___________ bonds, which it results in an conductor/semiconductor/insulator material.
b)Highest bands are called covalent/conduction/valence band. The forbidden bands, conversely, are named ______________.
c) As the temperature increases/decreases the doping level decreases/increases, resulting in a greater/lower value of ______________.
d)A delocalized is electron is shared by at least 1/2/3 atoms. The movement of these delocalized electron is called ______________
EXTRA) Draw the conductivity mechanism of one the three polymers discussed. Even though the mechanism is different, there is something in common. What is it?
Answers
- What are the three method of production of conductive polymers? And what are their limitations?
The chemical, electrochemical, and the photoelectrochemical. The chemical needs a high control in the process, since the reaction is very exothermic, which is releases a big amount of energy. The electrochemical limitation is related to the shape of the polymer that has the shape of the electrode, being necessary the posterior processing. Finally, the photoelectrochemical result a material that does not have good mechanical properties. - Why do most of polymers have a poor conductivity? And why do a conductive polymer have a great conductivity? [Hint: Your answer may have this words: covalent bond, band theory, band gap, delocalized electrons, resonance, dopants.]
Polymers are organic macromolecules and therefore having covalent bonds, which are directional bonds. Thus, the electrons are locked in these bonds, cannot being drift along the material, resulting in the poor conductivity. Moreover, according to the Band Theory, polymers have the band structure of an insulator, because have a big band gap. This theory is derived from the Schrödinger's Equation in a periodic potential, representing the lattice. When a polymer is doped, it arises charges in the polymer. For polymers with alternating double and single bonds, which was doped, has a movement of charge in the chain by resonance resulting in conductivity. - Draw the band structure of a polymer and a conductive polymer. Discuss what results this difference.
Figure 9 – Comparison of the Band Structure of a Common Polymer and a Conductive Polymer
When a polymer is doped, there are charges in it. Due to resonance the charge can drift through the chain, generating the conductivity. Due to the resonance contributors, the electron can occupy other energy levels. These new levels, that can be called polaronic bands, decreases the amount of energy that the electron needs to be excited to the conduction band, making these polymers as a semiconductor. - Circle or complete the blanks
a)Polymers are macro/micro molecules composed mostly by covalent bonds, which it results in an conductor/semiconductor/insulator material.
b)Highest bands are called covalent/conduction/valence band. The forbidden bands, conversely, are named _Band Gap_.
c) As the temperature increases/decreases the doping level decreases/increases, resulting in a greater/lower value of conductivity.
d)A delocalized is electron is shared by at least 1/2/3 atoms. The movement of these delocalized electron is called resonance.
EXTRA) Draw the conductivity mechanism of one the three polymers discussed. Even though the mechanism is different, there is something in common. What is it?
Taking the polyacetylene as an example:
With the dopants, charges are formed in the compound. This charges can drift through the chain by resonance, as shown above in the figure 10. The double bonds resonate in the direction of the carbocation - carbon with the positive charge - in order to stabilize it. With this, the positive charge can move freely. With an applied field, this charge can drift orderly in one direction resulting in a current. Due to this, the polyacetylene has a high value of conductivity. Even though the mechanism, the type of charge formed and the structure of the compounds are different, all of them is based in the resonance of the double bond.
Links
[a] http://chemwiki.ucdavis.edu/<wbr/>Theoretical_Chemistry/<wbr/>Chemical_Bonding/Covalent_<wbr/>Bonding
[b] http://chemwiki.ucdavis.edu/<wbr/>Theoretical_Chemistry/<wbr/>Chemical_Bonding/General_<wbr/>Principles_of_Chemical_<wbr/>Bonding/Band_Structure#<wbr/>Conductors.2C_Insulators_and_<wbr/>Semiconductors[c] http://chemwiki.ucdavis.edu/<wbr/>Textbook_Maps/Inorganic_<wbr/>Chemistry_Textbook_Maps/Map%<wbr/>3A_Housecroft/06%3A_<wbr/>Structures_and_energetics_of_<wbr/>metallic_and_ionic_solids/6.<wbr/>08%3A_Bonding_in_Metals_and_<wbr/>Semicondoctors/6.8A%3A_<wbr/>Electrical_Conductivity_and_<wbr/>Resistivity
[d] http://chemwiki.ucdavis.edu/<wbr/>Theoretical_Chemistry/<wbr/>Chemical_Bonding/Valence_Bond_<wbr/>Theory/Resonance[e] http://chemwiki.ucdavis.edu/<wbr/>Theoretical_Chemistry/<wbr/>Chemical_Bonding/Valence_Bond_<wbr/>Theory/Resonance/<wbr/>Delocalization_of_Electrons
References
[1]Bruice. Essential Organic Chemistry. 3rd ed. Santa Barbara: Pearson, 2012.
[2]Canevarolo. Science of Polymers. 2nd ed. Sao Paulo: Artliber, 2002.
[3]Callister. Materials Science and Engineering: An introduction – Seventh edition. LTC. 2007.
[4]Hummel, Electronic Properties of Materials, 3rd/4th Edition, Springer, 2001/2011.
[5]Hesketh & Misra. Conductive Polymers and Their Application. 2012
[7]Kasap, Principles of Electronic Materials and Devices, 3rd Edition, McGraw-Hill, 2000.
[8]Serway & Jewett. Principles of physics – Electromagnetism, 3rd Edition, Thomson, 2006.
[9]Street & Bredas. Polarons, Bipolarons, and Solitons in Conducting Polymers. Acc. Chemistry Res. 1985
[10]Skotheim & Reynolds. Handbook of Conducting Polymers. Conjugated Polymers: Processing and Application. 3ª Ed. CRC Press, 2007. Cap. 11, p. 1-33.
[11]Srilalitha , Jayaveera & Madhvendhra. The Effect of Dopant, Temperature, and Band Gap on Conductivity of Conducting Polymers. International Journal of Innovative Research in Science, Engineering and Technology Vol. 2, Issue 7, 2013.
[12]Ziadan. Conducting Polymers Application. New polymers for Special Application. Intech,2012.
Contributors and Attributions
Fernando Takashi da Rocha Arita - (Materials Science and Engineering - UC Davis)